The current status and clinical value of circulating tumor cells and circulating cell-free tumor DNA in bladder cancer
Introduction
With an estimated 430,000 newly diagnosed cases and over 165,000 deaths worldwide among both genders, urothelial carcinoma of the bladder (UCB) represents the 7th most common cancer worldwide and a significant burden of morbidity and mortality (1). The majority of patients present with non-muscle invasive UCB (NMIBC, non-muscle invasive bladder cancer) at initial diagnosis. Although recurrence rates in NMIBC are high with 50–70% and progression to muscle-invasive UCB (MIBC, muscle invasive bladder cancer) within 5 years is observed in about 15% of patients, most patients usually are eligible for bladder preserving treatment including transurethral resection and intravesical instillation therapies (2). However, up to a third of patients have muscle-invasive disease at primary diagnosis and up to 50% have recurrent high-grade non-muscle invasive disease or progression to muscle-invasive disease during their lifetime (3,4). Radical cystectomy (RC) with bilateral pelvic lymphadenectomy with or without perioperative chemotherapy is the standard of care in these patients (3).
Despite advances in the surgical and perioperative management, improvement of imaging techniques and progress in systemic therapies, outcomes virtually remain unchanged since decades and almost 50% of patients develop metastases and die from their disease within 5 years after surgery (5). Of importance a substantial number of patients demonstrate early progressive disease and develop metastases within the first 2 years after treatment with curative intent (6). This might be due to occult metastases growing from minimal residual disease (MRD), which remained undetectable even by modern, high resolution imaging procedures. Thus, biomarkers are urgently needed (I) to improve clinical staging as prerequisite for patient counseling and therapy guidance in UCB; (II) to detect MRD; (III) for predicting therapy response and subsequently prognosis and (IV) to enable patient stratification for multimodal treatment and therapy monitoring, respectively. Phenotypically similar tumors may harbor completely different molecular genotypes representing the individuality of each tumor and its host (7). Besides common histopathological parameters such as tumor stage and grade, genetic characterization and classification of UCB in different molecular subtypes has gained clinical interest (8). However, because of the strong heterogeneity of UCB and conflicting results of already applied targeted therapies which were based on genetic or gene expression profiling of tumor tissue, implications for targeted treatment still have to be optimized and validated in clinical trials (9). Several tissue and blood-based biomarkers have been investigated and hold the potential to unmask individual genomic, epigenetic, transcriptomic, and proteomic alterations that may explain the variable clinical course of disease (10). Biomarkers that may detect clinically relevant occult metastatic disease or MRD may help selecting patients best suited for multimodal therapy and sparing those patients, who are likely to be cured with local therapy alone, from the toxicity associated with unnecessary systemic therapy (11).
Likewise, in patients with metastatic or unresectable disease, therapeutic decisions ideally should be based on predictive biomarkers. Although standard platinum-based systemic chemotherapies yield initially in reasonable response rates, a significant number of patients progresses after approximately 1 year and the optimal subsequent systemic treatment remains unsettled since second-line agents demonstrated only limited activity (12). Administration of biologically targeted agents combined with systemic chemotherapy may represent an option for advanced, metastatic UCB patients with improved response rates. Nevertheless, systemic UCB chemotherapies often cause hematologic and non-hematologic side effects with serious grade 3/4 toxicities in over 50% of patients (13). Also of importance, new immunomodulatory therapies, which demonstrated reasonable response rates in several patients, still fail in a substantial percentage of patients and are causing enormous costs (14-16).
In this review article we summarize the current state, clinical application, potential and limitations of circulating tumor cells (CTCs) and circulating cell-free tumor DNA originating from blood as biomarkers in patients with different UCB stages.
Potential of liquid biopsy as biomarker
To date, decisions to treat cancer patients are mainly driven by characteristic features of the primary tumor and potentially its metastasis. For this reason, tumor tissue is evaluated by immunohistochemistry, fluorescence in situ hybridization (FISH), gene expression profiling or genomic analyses. In most cases, however, only an incomplete copy of the multitude of different facets of tumor cells can be reflected in daily routine. The situation is even more complicated when tumor disease progresses, individual tumor cell clones propagate and tumor cells acquire additional molecular aberrations over time and under treatment conditions. Identification of underlying driving forces and their elimination would require comprehensive evaluation of primary tumors, recurrences and metastases. However, restaging by evaluation of biopsies from metastases is restricted to selected easily accessible organs such as liver or skin and mostly impossible for other target organs. Thus, essential information for elimination of tumor cells in advanced stage patients is missing (17-20).
Unfortunately, conventional serum tumor markers are inaccurate and frequently fail because of low sensitivity and specificity. Accordingly, new biomarkers capable to real time record and monitor the actual disease state as well as to predict prognosis, therapy response and resistance detectable without invasive intervention are urgently needed in “precision medicine”. There is growing evidence that liquid biopsies have the potential to fill this gap (17-20).
For UCB urine intuitively seems to be the optimal resource, because of the direct contact to tumor tissue and tumor cell release (21). For that reason analyses of urine samples (i.e., urine cytology) are routinely performed in daily clinical practice. Urine cytology combined with FISH as well as determination of tumor-related antigens (bladder tumor antigens, NMP22) and of genomic aberrations in DNA isolated from urine has recently been improved mainly for diagnostic implications, but still lacks sufficient clinical validation (22,23). Suggested from a very recent study by Patel et al., mutant DNA detected in urine also has the potential to serve as biomarker for monitoring of neoadjuvant therapy (NT) (21). However, analyses of urinary samples will not be in the scope of our current review.
In this article, we focus on blood as liquid biopsy with special emphasis on CTCs and cell-free circulating tumor DNA (ctDNA) (Figure 1). Blood samples are easily accessible during the course of the disease and serial collection allows for long term observation of cancer patients. While CTCs have to be enriched from whole blood, buffy coats or enriched peripheral blood mononuclear cells immediately after blood drawing, ctDNA is isolated from serum or plasma samples which can be stored after collection until processing. Thus, both potential biomarkers have advantages and disadvantages and the most suitable one has to be chosen according to the question to be addressed and the particular study design (24,25).
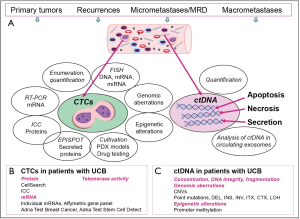
CTCs
CTCs are rare cells and especially in early tumor stages their concentration is very low thus requiring pre-enrichment to eliminate erythrocytes and the majority of leukocytes (26). Several enrichment methods relying either on physical properties such as cell size, plasticity, density or dielectrophoretic mobility or on the expression of antigens captured by high affinity magnetic particle-coated antibodies have been established yet. However, selecting the most effective one depending on the particular study design and planned down-stream analyses is challenging (26). Also for the detection of CTCs within the enriched cells manifold approaches are available and identification of tumor-cell specific transcripts by reverse transcriptase PCR (RT-PCR) or antigens by immunocytochemistry enable distinguishing CTCs from still contaminating blood cells (26,27). Because of the lack of tumor cell-specific markers, still epithelial cell properties represent the gold standard as surrogate markers to trace CTCs. Advantages and disadvantages of currently available detection platforms are discussed in several recent review articles (26-29).
Moreover, numerous accompanying approaches have been established for phenotypical and molecular characterization of CTCs to complement enumeration and quantification (Figure 1). Expression of therapeutically relevant proteins such as HER2 (human epidermal growth factor receptor), EGFR (epidermal growth factor receptor), ER (estrogen receptor), AR (androgen receptor) or PSMA (Prostate Specific Membrane Antigen) investigated by immunocytochemistry has been reported for CTCs derived from breast, colorectal and prostate cancer (30-35). Also, transcriptomic profiles were commonly described for enriched CTC populations (36,37), and even at single CTC level (38). Moreover, different approaches to explore genomic aberrations of CTCs have been established including FISH and PCR-based techniques. For single CTC analyses, propagation of DNA by WGA (whole genome amplification) is required, before mutational and CNV (copy number variations) analyses by Sanger sequencing, CGH (comparative genomic hybridization) and NGS (next generation sequencing) can be applied (35,39-41). Now clinical studies have to verify whether genomic aberrations of CTCs reflect the actual genomic landscape of the disease and therapy response or resistance, respectively, more accurately than genomic profiles of the corresponding primary tumors.
The successful isolation of viable CTCs has paved the way for a variety of down-stream investigations such as detection of secreted proteins, cultivation, xenograft and PDX (patient-derived xenografts) models which enable drug testing and further experiments to identify new therapeutic targets (Figure 1) (42-47).
CTCs in patients with UCB
Detection of CTCs by immunological methods
Meanwhile, several studies investigated the clinical relevance of CTC in UCB, however, the comparison of results from different groups is only feasible if similar detection techniques were applied (Table 1). The only standardized method available thus far is the CellSearch system, which has been cleared by the FDA (Food and Drug Administration) for the analysis of blood samples from patients with metastatic breast, prostate and colorectal cancer (88-90). In brief, in the first step CTCs are enriched from whole blood (either peripheral or from tumor-draining veins) by anti-EpCAM (epithelial cell adhesion molecule) antibodies coated to ferrofluid. In a second more specific step, identification of CTCs is performed by immunofluorescence with anti-keratin (KRT) antibodies and negativity for the common leukocyte antigen CD45. Subsequently, scanning of cells is carried out by an automated fluorescence microscope and suspicious images are presented in an image gallery for visual evaluation. High specificity and reproducibility of this system has been demonstrated in several multicenter studies (88,91,92). Although not yet included in the above-mentioned FDA approval, CTCs derived from patients with UCB can also be detected with the CellSearch system (Table 1). However, the numbers of enrolled patients are mostly low and no large multicenter clinical trials incorporating CTC analyses have been initiated, yet.
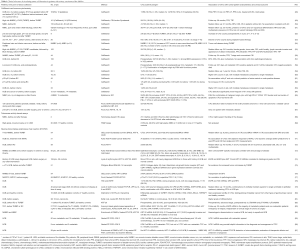
Full table
Using the Cell Search system, in UCB patients with non-metastatic disease CTCs were detected in about 20–30% of patients before surgical intervention or chemotherapy (Table 1) (48-53,55,57,59,61). The presence of CTCs correlated to FDG-PET-CT (2-deoxy-2-18F-fluoro-d-glucose positron emission tomography/computer tomography) imaging (48), but was not predictive for extra-vesical or node-positive disease (61). Interestingly, in most studies CTC detection was not associated with clinicopathological parameters.
In cohorts of patients with NMIBC including exclusively patients with high grade (G3) pT1 tumors, about 20% of patients harboured CTCs. In these studies, CTC detection was significantly associated with a reduced time to first recurrence and time to disease progression, defined as upstaging of the disease or appearance of distant metastases (49,55).
For patients with MIBC or recurrent high risk NMIBC treated with RC, the proof of CTCs had prognostic relevance regarding inferior recurrence-free, cancer-specific and overall survival (RFS, CSS, OS). The presence of CTCs was confirmed as independent predictive factor for early systemic disease and OS by multivariable analysis that adjusted for standard clinicopathological parameters (57,59). Of note, another study demonstrated that considering only patients who were spared from adjuvant chemotherapy after RC (Table 1), presence of CTCs prior to RC had an independent prognostic value predicting RFS, CSS and OS (50). The authors concluded that proof of CTCs potentially may identify patients in need of adjuvant chemotherapy and therefore may be a useful marker for patient counseling and decision-making. In contrast, in the same study, the presence of CTCs did not have prognostic relevance for RFS, CSS and OS, respectively, in patients who did receive adjuvant chemotherapy (50). This effect may either be a result of the potential of adjuvant chemotherapy on CTCs or due to the unfavorable general prognosis in UCB patients scheduled for adjuvant chemotherapy. In a further study the same group of authors found that CTCs are detectable in pure UCB and in UCB with variant histologies. CTCs still represented an independent prognostic factor for clinical outcome when results were adjusted for pure UCB or those with variant histology among other clinicopathological parameters (51).
A significantly higher number of CTCs was detected in patients with metastatic UCB ranging from 30% to 100% in different studies (Table 1) (48,53,58-60,63,64,93).
The CellSearch system only ensures detection of CTCs that are positive for EpCAM and KRT. Thus, tumor cells that have lost their epithelial features, e.g., in the course of epithelial-to mesenchymal transition (EMT) cannot be detected by this device. However, as evidenced recently by using the Epic Sciences platform, also KRT-negative CTCs are present in the circulation from UCB patients. Here, the malignant character of KRT-negative CTCs was confirmed by chromosomal aberrations detected by FISH and CNVs (65). Another study also reported usage of EpCAM-independent enrichment of CTCs by depletion of CD45-positive cells using magnetic cell separation and subsequent immunocytochemistry with anti-KRT antibodies. The CTC detection rate in this study was higher than those described in studies using the CellSearch system (Table 1) (66). Lower detection rates were observed when applying anti-KRT antibodies in ICC on PBMCs enriched by density gradient centrifugation (67). Moreover, application of assays based on the activity of the enzyme telomerase that is thought to be expressed only in tumor and not in normal cells might help to identify CTCs that have down-regulated epithelial cell properties (Table 1) (68,69).
Currently efforts are spent for establishing phenotypical markers valuable to stratify UCB patients for individualized targeted therapies. In this context the detection of HER2 expression of CTCs has to be mentioned. Discordances detected between the HER2 status of CTCs and that of the corresponding primary tumors suggest that a subgroup of patients with HER2-positive CTCs likely profits from a HER2 targeting therapy (57). Although still not conclusively shown in large studies treatment of HER2-positive MIBC, who were not candidates for RC, by daily radiation combined with paclitaxel and trastuzumab revealed to be an effective strategy with high completion rate and moderate toxicity (94). On the other site, the presence of HER2-negative CTCs in patients with HER2-positive corresponding primary tumors might be responsible for the failure of HER2-targeting therapies in a considerable number of patients (57). In patients with metastatic UCB, a high concordance of the HER2 status between CTCs and primary tumors could be observed (93).
Moreover, there is an urgent necessity to identify biomarkers predictive of response to immune checkpoint inhibitors which have already been FDA-approved for the treatment of patients with metastatic UCB (95). Anantharaman et al. were the first to display programmed cell death-ligand 1 (PD-L1) expression on metastatic UCB-derived CTCs (65), however clinical value of PD-L1 analysis on CTCs remains to be conclusively shown in large clinical immune checkpoint inhibitor studies. Interestingly, PD-L1 expression of KRT-positive and KRT-negative CTCs has been demonstrated in this study.
Detection of CTCs by RT-PCR
Besides methods allowing also assessment of morphological characteristics and gene expression levels of individual CTCs, assays to detect CTCs by tumor cell specific transcripts from enriched CTCs have been established. Also here, initial enrichment of CTCs is required and commonly achieved by anti-EpCAM antibodies coupled to magnetic beads (70,71,73) or by marker-independent approaches such as density gradient centrifugation (77). Moreover, RNA was isolated from whole blood with preceding red blood lysis in some other studies (Table 1) (74,79). However, standardized assays and clinically validated cut-off values are still missing.
Once enriched, evidence of CTCs will be provided by highly sensitive RT-PCR approaches and quantification of tumor-cell-specific transcripts occurs by quantitative RT-PCR. The extraordinarily high sensitivity to trace even low abundant tumor cell transcripts might be accompanied by less specificity due to very low intensity or illegitimate expression of the gene of interest in contaminating leukocytes (96,97). Nevertheless, there are a remarkable number of studies demonstrating clinical value of CTCs detected by RT-PCR, e.g., in breast, prostate and lung cancer. In these studies either individual tumor cell transcripts or gene expression profiles were detected (17,98-102).
Table 1 summarizes results for CTC detection by RT-PCR obtained for UCB patients. Early studies for mainly small numbers of patients aimed to detect transcripts specific for KRT19 and UPK II (uroplakin II) demonstrated that CTCs originating from UCB can support detection of metastasis including micrometastases, evaluation of therapeutic effects and prediction of prognosis (85-87). KRT20-specific transcripts expressed by luminal subtypes of UCB revealed to be indicative of hematogenous tumor cell dissemination in MIBC, but not in superficial tumors (84) or prior to transurethral resection of the bladder tumor (TURBT) for advanced tumor stage (82). Studies published by Leotsakos et al. and Gazzaniga et al. (2001 and 2005) provided evidence for prognostic relevance of EGFR, KRT19, 20, UPKII and TNC (tenascin C) transcripts (74,78,83). Thus, detection of EGFR and TNC mRNAs was associated with reduced DFS and RFS for UCB patients. Osman et al. showed that the combined detection of different transcripts such as UPKI, II and EGFR or the examination of gene expression profiles by Affymetrix microchips was superior to single urothelial or epithelial markers in detecting CTCs (79,80). In patients with NMIBC, KRT8-, and BRC5 (survivin)-specific transcripts were predictive of reduced DFS (76) and DFS and CSS (70), respectively.
Applying the commercially available Adna Test Breast Cancer, or stem cell Test for blood samples from NMIBC and non-metastatic or metastatic MICB, Todenhöfer et al. assumed that epithelial cell-, but also stem cell- and EMT-specific transcripts are indicative for the clinical stage of the disease (73). Moreover, the prevalence of KRT7 mRNA detected before RC was associated with tumor and lymph node state, with increased risk of recurrence and independently with reduced CSS and OS of CSS of UCB patients (72). However, although no association of CTCs detected with the Adna Test Prostate Cancer—applied for UCB patients—with objective response to treatment of metastatic UCB (methotrexate, vinblastine, doxorubicin, cisplatin) could be observed, dynamic changes of CTCs were more predictive for progression-free survival (PFS) and OS than single point measurements (71).
In conclusion, prognostic relevance of CTC detection by RT-PCR could be demonstrated in several studies on still small patient cohorts. Application of those tests in large multi-centric studies is hindered by the low stability of RNA complicating shipment and storage of samples. However, similar to CellSave tubes for CellSearch strong efforts are made to develop blood-drawing tubes ensuring shipment and storage of blood samples for RT-PCR analyses without losing significant information. Moreover, from the variety of assay formats and UCB-associated transcripts the best suited have to be chosen to establish a standardized approach using a pre-defined UCB-specific panel of transcripts. Then multi-center clinical trials for monitoring systemic therapies can be initiated with serial blood investigations during follow up observations of the patients.
Release of tumor cells by surgical interventions
There is growing interest in addressing the question whether surgical or other manipulations on tumor tissue are accompanied by tumor cell release which gives rise to later development of recurrences or metastasis.
For patients with NMIBC, conflicting results from only three studies enrolling small numbers of patients are available. While tumor cell release was observed by Blaschke et al. and Engilbertsson et al. applying the CellSearch system (Table 1) (52,54), an RT-PCR-based study did not provide evidence for tumor cell release by TURBT (75). Conclusively, only serial CTC measurements at defined time points within prospective long term-follow up studies on large patient cohorts can uncover whether increased CTC counts by surgical intervention or other tumor manipulations are associated with an increased risk of disease recurrence and metastases. Additionally, strong efforts have to be done by molecular approaches and functional studies to identify metastasis-initiating cells among these tumor cells as well as to discover potential metastatic seeding of released tumor cells. Importantly, future studies will investigate if improvements in operation techniques might have the ability to avoid tumor cell spreading.
Detection of circulating cell-free tumor DNA (ctDNA) in patients with UCB
During recent years, circulating cell-free tumor DNA (ctDNA) has gained attraction as candidate biomarker with potential to improve diagnostics, detection of MRD and therapeutic monitoring of tumor disease. Typical size of cfDNA fragments is 160 to 180 base pairs similar to the length of nucleosome-protected DNA in apoptotic cells (103). Although DNA can be released from all kinds of cells by apoptosis, necrosis or even by active secretion and therefore is detectable in different body fluids such as blood, urine, sputum, or cerebrospinal fluid, results from different studies indicate that the concentration of cell-free DNA is higher in tumor patients compared to healthy individuals (103). However, increase of concentration can also be due to inflammatory processes, exercising or non-tumor diseases, thus requiring additional markers to discriminate between normal and tumor-derived DNA (103). As genomic or epigenetic aberrations are key features of tumor cells, covering somatic alterations in cell-free DNA (cfDNA) is indicative of the presence of cell-free tumor DNA (ctDNA) (Figure 1). In the pre-NGS era of circulating DNA investigations, instability in frequent microsatellite markers was used to identify loss of heterozygosity (LOH) as proof of tumor cell origin or hypermethylation of promoter regions regulating tumor suppressor or other tumor-related genes in cell-free DNA (104). Later on, technological advances in PCR and NGS approaches prepared the ground for the present capability to comprehensively analyse tumor-originating DNA accounting for less than 0.1% of total cfDNA in patients with early cancer and up to 10% in advanced stage cancer in plasma or serum (25,103,105,106).
Compared to CTCs, investigation of ctDNA has the advantage that plasma/serum samples can be long-term stored and easily be collected until final analysis, making ctDNA analyses very attractive for multicenter studies with complex and standardized assays centrally applied. The challenge of ctDNA research is to find marker alterations out of the diversity of potential genomic aberrations suitable to follow the individual tumor patient. New techniques including massive parallel NGS to explore high numbers of genes and sensitive PCR approaches to trace single mutant molecules are established, however are not amenable for daily routine applications yet (25,103).
To date, personalized ctDNA assays based on comprehensive genomic evaluation of the primary tumors are in the centre of attention and have already been approved in smaller pilot studies (107-109). Mostly performed by highly sensitive digital droplet PCR (ddPCR), personalized assays enable direct reflection of individual tumor-specific aberrations in plasma or serum samples. Moreover, circulating extracellular vesicles (exosomes, Figure 1) suggested to carry and transfer tumor-derived DNA (110) hold promise as informative biomarkers in cancer patients (111), but will not be discussed in this article.
Detection of ctDNA by epigenetic alterations in patients with UCB
Tumor suppressor genes can be inactivated by hypermethylation of CpG islands in their promoter regions (104). Using methylation-sensitive/specific PCR (MSP), aberrant DNA methylation of promoter regions from a multitude of tumor-associated genes in serum-DNA could be detected in several studies (Table 2). The most prominent analyzed gene was p16INK4a, encoding a tumor-suppressing cyclin-dependent kinase inhibitor which is frequently inactivated in cancer of various organs including bladder by mutations, LOH or promoter hypermethylation (126,127). As opposed to CTCs, detection of promoter hypermethylation in circulating cfDNA was frequently associated with higher tumor stages and poor differentiation (113,117,119). However, Hauser et al. did not find any association of promoter methylation in cfDNA with clinicopathological parameters (115). Instead, methylation levels at each site and number of methylated genes was higher in UCB patients compared to healthy controls (115) and methylation in promoter regions from selected genes distinguished UCB patients from healthy controls (119). Moreover, proof of methylation in the promoter regions of H-Cadherin-13 (CDH13) (117) and Protocadherin-17 (PCDH17) (113) genes were of prognostic relevance for a shorter OS.
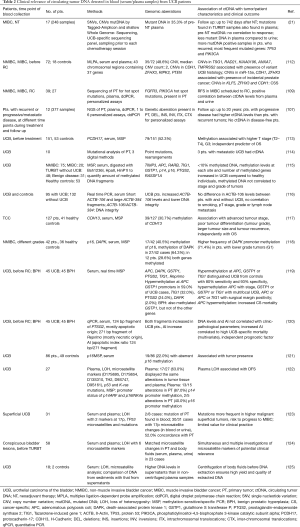
Full table
Profiling of genomic aberrations in plasma or serum-derived circulating DNA isolated from patients with UCB
As evidenced by microsatellite-based PCR analysis, LOH was frequently detected in serum and plasma collected from patients with UCB (Table 2). Frequently affected microsatellites were located on chromosomes 17p and 9p obviously influencing the activity of tumor suppressor genes such as p53 and p16/p14 (122,123). Reduced DFS (122) and higher risk of NMIBC for progression to MIBC (123) was associated with LOH in these microsatellite markers (Table 2).
Technical advances mainly in NGS- and PCR-based techniques have provoked enormous progress towards clinical application of ctDNA research findings (103). Very recently, Patel et al. and Soave et al. identified CNVs and mutations in tumor-related genes by NGS and multiplex ligation-dependent probe amplification (MPLA), respectively in ctDNA from patients with non-metastatic UCB (21,112). Moreover, frequently mutated genes were TP53 and PIK3CA, known to inactivate the tumor suppressor TP53 and to activate the oncoprotein PIK3CA, respectively (21).
In two retrospective studies Birkenkamp-Demtröder et al. and Christensen et al. established personalized assays based on comprehensive NGS of the corresponding primary tumors (107,109) for ctDNA analysis in plasma samples. While the first study focused on hot spot mutations in TP53 and PIK3CA and found ctDNA associated with a reduced DFS in MIBC (109), the other study developed personalized assays according to genomic aberrations in the primary tumors and absent in germline DNA uncovered by three different NGS approaches. Here, they analyzed deletions, insertions, inversions, as well as intra- and inter chromosomal translocations evident in different chromosomal regions and followed the 12 patients with a total of 377 samples for up to 20 years (107). Higher levels of ctDNA detected in plasma before progression of disease were found in patients with progressive disease to MIBC, when compared to patients with recurrent NMIBC. ctDNA was no longer detectable in patients with no proof of disease during follow up (107) suggesting that monitoring of tumor progression by serial performing of personalized assays is feasible. Unexpectedly, plasma samples of two patients with progression of disease lacked ctDNA, thus ctDNA did not reflect tumor burden and actual state of the disease (107). Whether phagocytosis or upregulated DNA degradation mechanisms are responsible for elimination of ctDNA as postulated by Heidary et al. for a similar finding in metastatic breast cancer (128) remains to be elucidated. Moreover, clinical validation of these results on larger patient cohorts within prospective clinical trials with defined endpoints is urgently needed.
Summary and outlook
Assays detecting and characterizing CTCs and ctDNA are valuable tools in view of clinical tumor staging and prediction of clinical outcomes for patients with UCB. CTCs detected in patients with high-risk NMIBC and MIBC prior to surgical interventions are significantly associated with disease recurrence and inferior survival. Furthermore, there is some evidence that CTCs may help identifying patients in need of systemic chemotherapy. However, interventional or randomized studies based on CTC stratification are needed for further clarification, if this biomarker is ready for prime time in patient guidance regarding multimodal therapies.
Besides enumeration, phenotypical and molecular characterization of CTCs has gained attention to identify and validate new markers intended to get implemented in targeted therapies. Pilot studies to validate HER2 and PD-L1 expression on CTCs have been conducted and now have to be transferred to clinical studies. Although not yet applied for UCB, genetic, epigenetic and transcriptomic approaches at single CTC level have been established and are amenable to identify new therapeutic targets and to get deeper insights into tumor cell heterogeneity. A major disadvantage of CTCs as biomarker is their low prevalence in patients with early stage UCB and also the fact that there are a considerable number of patients with advanced UCB unexpectedly lacking proof of CTCs. Although standardized and automated, the CellSearch assay does not have the ability to find CTCs that have lost epithelial cell features in the course of EMT. Hence, recent technical advances in CTC approaches established to detect also mesenchymal-like CTCs mainly in breast cancer have to be exploited also for UCB.
To date, there is a strong discussion whether CTCs or ctDNA represent the better liquid biopsy tool. Indeed, ctDNA can be easily isolated from stored plasma or serum samples and if comprehensively validated has the potential to better mirror tumor cell heterogeneity and real time reflect all tumor sub-clones and hence the genomic landscape of the tumor disease. However, costs for whole exome/genome analyses are still high and personalized assays using individual markers or gene panels are dependent on previous analyses of corresponding tumor tissues, which are only rarely available in advanced tumor stages (25).
In conclusion, for UCB large-scale prospective clinical or randomized studies with defined endpoints are urgently warranted validating the promising results of liquid biopsies from available smaller observational studies. Due to complicated accrual issues in UCB trials in the past, it may be useful to include liquid biopsies in currently designed UCB interventional studies to shed more light on these blood-based biomarkers.
Acknowledgements
None.
Footnote
Conflicts of Interest: The authors have no conflicts of interest to declare.
References
- Torre LA, Bray F, Siegel RL, et al. Global cancer statistics, 2012. CA Cancer J Clin 2015;65:87-108. [Crossref] [PubMed]
- Babjuk M, Böhle A, Burger M, et al. EAU Guidelines on Non-Muscle-invasive Urothelial Carcinoma of the Bladder: Update 2016. Eur Urol 2017;71:447-61. [Crossref] [PubMed]
- Witjes JA, Compérat E, Cowan NC, et al. EAU guidelines on muscle-invasive and metastatic bladder cancer: summary of the 2013 guidelines. Eur Urol 2014;65:778-92. [Crossref] [PubMed]
- Babjuk M, Burger M, Zigeuner R, et al. EAU guidelines on non-muscle-invasive urothelial carcinoma of the bladder: update 2013. Eur Urol 2013;64:639-53. [Crossref] [PubMed]
- Weisbach L, Dahlem R, Simone G, et al. Lymph node dissection during radical cystectomy for bladder cancer treatment: considerations on relevance and extent. Int Urol Nephrol 2013;45:1561-7. [Crossref] [PubMed]
- Rink M, Lee DJ, Kent M, et al. Predictors of cancer-specific mortality after disease recurrence following radical cystectomy. BJU Int 2013;111:E30-6. [Crossref] [PubMed]
- Rink M, Shariat SF, Soave A. Liquid biopsies in bladder cancer-did we find the Holy Grail for biomarker analyses? Transl Androl Urol 2016;5:980-3. [Crossref] [PubMed]
- Seiler R, Ashab HA, Erho N, et al. Impact of Molecular Subtypes in Muscle-invasive Bladder Cancer on Predicting Response and Survival after Neoadjuvant Chemotherapy. Eur Urol 2017;72:544-54. [Crossref] [PubMed]
- Kamat AM, Hahn NM, Efstathiou JA, et al. Bladder cancer. Lancet 2016;388:2796-810. [Crossref] [PubMed]
- Rink M, Cha EK, Green D, et al. Biomolecular predictors of urothelial cancer behavior and treatment outcomes. Curr Urol Rep 2012;13:122-35. [Crossref] [PubMed]
- Soave A, Riethdorf S, Pantel K, et al. Do circulating tumor cells have a role in deciding on adjuvant chemotherapy after radical cystectomy? Curr Urol Rep 2015;16:46. [Crossref] [PubMed]
- Oing C, Rink M, Oechsle K, et al. Second Line Chemotherapy for Advanced and Metastatic Urothelial Carcinoma: Vinflunine and Beyond-A Comprehensive Review of the Current Literature. J Urol 2016;195:254-63. [Crossref] [PubMed]
- Soave A, Engel O, Von Amsberg G, et al. Management of advanced bladder cancer in the era of targeted therapies. Minerva Urol Nefrol 2015;67:103-15. [PubMed]
- Dobbin KK, Cesano A, Alvarez J, et al. Validation of biomarkers to predict response to immunotherapy in cancer: Volume II - clinical validation and regulatory considerations. J Immunother Cancer 2016;4:77. [Crossref] [PubMed]
- Hakenberg OW. Nivolumab for the treatment of bladder cancer. Expert Opin Biol Ther 2017;17:1309-15. [Crossref] [PubMed]
- Masucci GV, Cesano A, Hawtin R, et al. Validation of biomarkers to predict response to immunotherapy in cancer: Volume I - pre-analytical and analytical validation. J Immunother Cancer 2016;4:76. [Crossref] [PubMed]
- Pantel K, Alix-Panabières C, Riethdorf S. Cancer micrometastases. Nat Rev Clin Oncol 2009;6:339-51. [Crossref] [PubMed]
- Alix-Panabières C, Pantel K. Real-time liquid biopsy: circulating tumor cells versus circulating tumor DNA. Ann Transl Med 2013;1:18. [PubMed]
- Alix-Panabières C, Pantel K. Clinical Applications of Circulating Tumor Cells and Circulating Tumor DNA as Liquid Biopsy. Cancer Discov 2016;6:479-91. [Crossref] [PubMed]
- Pantel K, Alix-Panabières C. Liquid biopsy: Potential and challenges. Mol Oncol 2016;10:371-3. [Crossref] [PubMed]
- Patel KM, van der Vos KE, Smith CG, et al. Association Of Plasma And Urinary Mutant DNA With Clinical Outcomes In Muscle Invasive Bladder Cancer. Sci Rep 2017;7:5554. [Crossref] [PubMed]
- Bell MD, Yafi FA, Brimo F, et al. Prognostic value of urinary cytology and other biomarkers for recurrence and progression in bladder cancer: a prospective study. World J Urol 2016;34:1405-9. [Crossref] [PubMed]
- Zuiverloon TC, Beukers W, van der Keur KA, et al. Combinations of urinary biomarkers for surveillance of patients with incident nonmuscle invasive bladder cancer: the European FP7 UROMOL project. J Urol 2013;189:1945-51. [Crossref] [PubMed]
- Lowes LE, Bratman SV, Dittamore R, et al. Circulating Tumor Cells (CTC) and Cell-Free DNA (cfDNA) Workshop 2016: Scientific Opportunities and Logistics for Cancer Clinical Trial Incorporation. Int J Mol Sci 2016.17. [PubMed]
- Pantel K. Blood-Based Analysis of Circulating Cell-Free DNA and Tumor Cells for Early Cancer Detection. PLoS Med 2016;13:e1002205. [Crossref] [PubMed]
- Joosse SA, Gorges TM, Pantel K. Biology, detection, and clinical implications of circulating tumor cells. EMBO Mol Med 2015;7:1-11. [Crossref] [PubMed]
- Alix-Panabières C, Pantel K. Technologies for detection of circulating tumor cells: facts and vision. Lab Chip 2014;14:57-62. [Crossref] [PubMed]
- Chinen LT, Abdallah EA, Braun AC, et al. Circulating Tumor Cells as Cancer Biomarkers in the Clinic. Adv Exp Med Biol 2017;994:1-41. [Crossref] [PubMed]
- Kowalik A, Kowalewska M, Góźdź S. Current approaches for avoiding the limitations of circulating tumor cells detection methods-implications for diagnosis and treatment of patients with solid tumors. Transl Res 2017;185:58-84.e15. [Crossref] [PubMed]
- Riethdorf S, Müller V, Zhang L, et al. Detection and HER2 expression of circulating tumor cells: prospective monitoring in breast cancer patients treated in the neoadjuvant GeparQuattro trial. Clin Cancer Res 2010;16:2634-45. [Crossref] [PubMed]
- Fehm T, Müller V, Aktas B, et al. HER2 status of circulating tumor cells in patients with metastatic breast cancer: a prospective, multicenter trial. Breast Cancer Res Treat 2010;124:403-12. [Crossref] [PubMed]
- Gasch C, Bauernhofer T, Pichler M, et al. Heterogeneity of epidermal growth factor receptor status and mutations of KRAS/PIK3CA in circulating tumor cells of patients with colorectal cancer. Clin Chem 2013;59:252-60. [Crossref] [PubMed]
- Babayan A, Hannemann J, Spotter J, et al. Heterogeneity of estrogen receptor expression in circulating tumor cells from metastatic breast cancer patients. PLoS One 2013;8:e75038. [Crossref] [PubMed]
- Gorges TM, Riethdorf S, von Ahsen O, et al. Heterogeneous PSMA expression on circulating tumor cells: a potential basis for stratification and monitoring of PSMA-directed therapies in prostate cancer. Oncotarget 2016;7:34930-41. [Crossref] [PubMed]
- Alix-Panabières C, Pantel K. Characterization of single circulating tumor cells. FEBS Lett 2017;591:2241-50. [Crossref] [PubMed]
- Sieuwerts AM, Kraan J, Bolt-de Vries J, et al. Molecular characterization of circulating tumor cells in large quantities of contaminating leukocytes by a multiplex real-time PCR. Breast Cancer Res Treat 2009;118:455-68. [Crossref] [PubMed]
- Sieuwerts AM, Mostert B, Bolt-de Vries J, et al. mRNA and microRNA expression profiles in circulating tumor cells and primary tumors of metastatic breast cancer patients. Clin Cancer Res 2011;17:3600-18. [Crossref] [PubMed]
- Gorges TM, Kuske A, Röck K, et al. Accession of Tumor Heterogeneity by Multiplex Transcriptome Profiling of Single Circulating Tumor Cells. Clin Chem 2016;62:1504-15. [Crossref] [PubMed]
- Heitzer E, Auer M, Gasch C, et al. Complex tumor genomes inferred from single circulating tumor cells by array-CGH and next-generation sequencing. Cancer Res 2013;73:2965-75. [Crossref] [PubMed]
- Polzer B, Medoro G, Pasch S, et al. Molecular profiling of single circulating tumor cells with diagnostic intention. EMBO Mol Med 2014;6:1371-86. [Crossref] [PubMed]
- Babayan A, Alawi M, Gormley M, et al. Comparative study of whole genome amplification and next generation sequencing performance of single cancer cells. Oncotarget 2016;8:56066-80. [PubMed]
- Baccelli I, Schneeweiss A, Riethdorf S, et al. Identification of a population of blood circulating tumor cells from breast cancer patients that initiates metastasis in a xenograft assay. Nat Biotechnol 2013;31:539-44. [Crossref] [PubMed]
- Williams ES, Rodriguez-Bravo V, Chippada-Venkata U, et al. Generation of Prostate Cancer Patient Derived Xenograft Models from Circulating Tumor Cells. J Vis Exp 2015.53182. [PubMed]
- Cayrefourcq L, Mazard T, Joosse S, et al. Establishment and characterization of a cell line from human circulating colon cancer cells. Cancer Res 2015;75:892-901. [Crossref] [PubMed]
- Pantel K, Alix-Panabières C. Cell lines from circulating tumor cells. Oncoscience 2015;2:815-6. [PubMed]
- Alix-Panabières C, Pantel K. Liquid biopsy in cancer patients: advances in capturing viable CTCs for functional studies using the EPISPOT assay. Expert Rev Mol Diagn 2015;15:1411-7. [Crossref] [PubMed]
- Maheswaran S, Haber DA. Ex Vivo Culture of CTCs: An Emerging Resource to Guide Cancer Therapy. Cancer Res 2015;75:2411-5. [Crossref] [PubMed]
- Abrahamsson J, Aaltonen K, Engilbertsson H, et al. Circulating tumor cells in patients with advanced urothelial carcinoma of the bladder: Association with tumor stage, lymph node metastases, FDG-PET findings, and survival. Urol Oncol 2017. [Epub ahead of print]. [Crossref] [PubMed]
- Busetto GM, Ferro M, Del Giudice F, et al. The Prognostic Role of Circulating Tumor Cells (CTC) in High-risk Non-muscle-invasive Bladder Cancer. Clin Genitourin Cancer 2017;15:e661-6. [Crossref] [PubMed]
- Soave A, Riethdorf S, Dahlem R, et al. A nonrandomized, prospective, clinical study on the impact of circulating tumor cells on outcomes of urothelial carcinoma of the bladder patients treated with radical cystectomy with or without adjuvant chemotherapy. Int J Cancer 2017;140:381-9. [Crossref] [PubMed]
- Soave A, Riethdorf S, Dahlem R, et al. Detection and oncological effect of circulating tumour cells in patients with variant urothelial carcinoma histology treated with radical cystectomy. BJU Int 2017;119:854-61. [Crossref] [PubMed]
- Blaschke S, Koenig F, Schostak M. Hematogenous Tumor Cell Spread Following Standard Transurethral Resection of Bladder Carcinoma. Eur Urol 2016;70:544-5. [Crossref] [PubMed]
- Winters B, James A, Lee J, et al. Chemotherapeutic effects on circulating tumor cells in bladder cancer. Int J Urol 2015;22:612-3. [Crossref] [PubMed]
- Engilbertsson H, Aaltonen KE, Bjornsson S, et al. Transurethral bladder tumor resection can cause seeding of cancer cells into the bloodstream. J Urol 2015;193:53-7. [Crossref] [PubMed]
- Gazzaniga P, de Berardinis E, Raimondi C, et al. Circulating tumor cells detection has independent prognostic impact in high-risk non-muscle invasive bladder cancer. Int J Cancer 2014;135:1978-82. [Crossref] [PubMed]
- Gazzaniga P, Gradilone A, de Berardinis E, et al. Prognostic value of circulating tumor cells in nonmuscle invasive bladder cancer: a CellSearch analysis. Ann Oncol 2012;23:2352-6. [Crossref] [PubMed]
- Rink M, Chun FK, Dahlem R, et al. Prognostic role and HER2 expression of circulating tumor cells in peripheral blood of patients prior to radical cystectomy: a prospective study. Eur Urol 2012;61:810-7. [Crossref] [PubMed]
- Flaig TW, Wilson S, van Bokhoven A, et al. Detection of circulating tumor cells in metastatic and clinically localized urothelial carcinoma. Urology 2011;78:863-7. [Crossref] [PubMed]
- Rink M, Chun FK, Minner S, et al. Detection of circulating tumour cells in peripheral blood of patients with advanced non-metastatic bladder cancer. BJU Int 2011;107:1668-75. [Crossref] [PubMed]
- Okegawa T, Hayashi K, Hara H, et al. Immunomagnetic quantification of circulating tumor cells in patients with urothelial cancer. Int J Urol 2010;17:254-8. [Crossref] [PubMed]
- Guzzo TJ, McNeil BK, Bivalacqua TJ, et al. The presence of circulating tumor cells does not predict extravesical disease in bladder cancer patients prior to radical cystectomy. Urol Oncol 2012;30:44-8. [Crossref] [PubMed]
- Karl A, Tritschler S, Hofmann S, et al. Perioperative search for circulating tumor cells in patients undergoing radical cystectomy for bladder cancer. Eur J Med Res 2009;14:487-90. [Crossref] [PubMed]
- Gallagher DJ, Milowsky MI, Ishill N, et al. Detection of circulating tumor cells in patients with urothelial cancer. Ann Oncol 2009;20:305-8. [Crossref] [PubMed]
- Naoe M, Ogawa Y, Morita J, et al. Detection of circulating urothelial cancer cells in the blood using the CellSearch System. Cancer 2007;109:1439-45. [Crossref] [PubMed]
- Anantharaman A, Friedlander T, Lu D, et al. Programmed death-ligand 1 (PD-L1) characterization of circulating tumor cells (CTCs) in muscle invasive and metastatic bladder cancer patients. BMC Cancer 2016;16:744. [Crossref] [PubMed]
- Meye A, Bilkenroth U, Schmidt U, et al. Isolation and enrichment of urologic tumor cells in blood samples by a semi-automated CD45 depletion autoMACS protocol. Int J Oncol 2002;21:521-30. [PubMed]
- Desgrandchamps F, Teren M, Dal Cortivo L, et al. The effects of transurethral resection and cystoprostatectomy on dissemination of epithelial cells in the circulation of patients with bladder cancer. Br J Cancer 1999;81:832-4. [Crossref] [PubMed]
- Ju M, Kao GD, Steinmetz D, et al. Application of a telomerase-based circulating tumor cell (CTC) assay in bladder cancer patients receiving postoperative radiation therapy: a case study. Cancer Biol Ther 2014;15:683-7. [Crossref] [PubMed]
- Soria JC, Morat L, Durdux C, et al. The molecular detection of circulating tumor cells in bladder cancer using telomerase activity. J Urol 2002;167:352-6. [Crossref] [PubMed]
- Nicolazzo C, Busetto GM, Del Giudice F, et al. The long-term prognostic value of survivin expressing circulating tumor cells in patients with high-risk non-muscle invasive bladder cancer (NMIBC). J Cancer Res Clin Oncol 2017;143:1971-6. [Crossref] [PubMed]
- Fina E, Necchi A, Giannatempo P, et al. Clinical Significance of Early Changes in Circulating Tumor Cells from Patients Receiving First-Line Cisplatin-Based Chemotherapy for Metastatic Urothelial Carcinoma. Bladder Cancer 2016;2:395-403. [Crossref] [PubMed]
- Pagliarulo V, Ancona P, Petitti T, et al. Detection and Clinical Significance of Circulating Tumor Cells in Patients Undergoing Radical Cystectomy for Urothelial Bladder Cancer. Clin Genitourin Cancer 2017;15:455-62. [Crossref] [PubMed]
- Todenhöfer T, Hennenlotter J, Dorner N, et al. Transcripts of circulating tumor cells detected by a breast cancer-specific platform correlate with clinical stage in bladder cancer patients. J Cancer Res Clin Oncol 2016;142:1013-20. [Crossref] [PubMed]
- Leotsakos I, Dimopoulos P, Gkioka E, et al. Detection of circulating tumor cells in bladder cancer using multiplex PCR assays. Anticancer Res 2014;34:7415-24. [PubMed]
- Antoniewicz AA, Paziewska A, Mikula M, et al. Lack of evidence for increased level of circulating urothelial cells in the peripheral blood after transurethral resection of bladder tumors. Int Urol Nephrol 2012;44:761-7. [Crossref] [PubMed]
- Gradilone A, Petracca A, Nicolazzo C, et al. Prognostic significance of survivin-expressing circulating tumour cells in T1G3 bladder cancer. BJU Int 2010;106:710-5. [Crossref] [PubMed]
- Ribal MJ, Mengual L, Marin M, et al. Molecular staging of bladder cancer with RT-PCR assay for CK20 in peripheral blood, bone marrow and lymph nodes: comparison with standard histological staging. Anticancer Res 2006;26:411-9. [PubMed]
- Gazzaniga P, Nofroni I, Gandini O, et al. Tenascin C and epidermal growth factor receptor as markers of circulating tumoral cells in bladder and colon cancer. Oncol Rep 2005;14:1199-202. [PubMed]
- Osman I, Kang M, Lee A, et al. Detection of circulating cancer cells expressing uroplakins and epidermal growth factor receptor in bladder cancer patients. Int J Cancer 2004;111:934-9. [Crossref] [PubMed]
- Osman I, Bajorin DF, Sun TT, et al. Novel blood biomarkers of human urinary bladder cancer. Clin Cancer Res 2006;12:3374-80. [Crossref] [PubMed]
- Kinjo M, Okegawa T, Horie S, et al. Detection of circulating MUC7-positive cells by reverse transcription-polymerase chain reaction in bladder cancer patients. Int J Urol 2004;11:38-43. [Crossref] [PubMed]
- Retz M, Lehmann J, Roder C, et al. Cytokeratin-20 reverse-transcriptase polymerase chain reaction as a new tool for the detection of circulating tumor cells in peripheral blood and bone marrow of bladder cancer patients. Eur Urol 2001;39:507-15. [Crossref] [PubMed]
- Gazzaniga P, Gandini O, Giuliani L, et al. Detection of epidermal growth factor receptor mRNA in peripheral blood: a new marker of circulating neoplastic cells in bladder cancer patients. Clin Cancer Res 2001;7:577-83. [PubMed]
- Fujii Y, Kageyama Y, Kawakami S, et al. Detection of disseminated urothelial cancer cells in peripheral venous blood by a cytokeratin 20-specific nested reverse transcriptase-polymerase chain reaction. Jpn J Cancer Res 1999;90:753-7. [Crossref] [PubMed]
- Li SM, Zhang ZT, Chan S, et al. Detection of circulating uroplakin-positive cells in patients with transitional cell carcinoma of the bladder. J Urol 1999;162:931-5. [Crossref] [PubMed]
- Yuasa T, Yoshiki T, Isono T, et al. Expression of transitional cell-specific genes, uroplakin Ia and II, in bladder cancer: detection of circulating cancer cells in the peripheral blood of metastatic patients. Int J Urol 1999;6:286-92. [Crossref] [PubMed]
- Kaneda T, Hoshi S, Mao H, et al. Detection of urogenital malignant cells in the peripheral blood by nested RT-PCR using keratin 19 mRNA. Nihon Hinyokika Gakkai Zasshi 1998;89:33-42. [Crossref] [PubMed]
- Cristofanilli M, Budd GT, Ellis MJ, et al. Circulating tumor cells, disease progression, and survival in metastatic breast cancer. N Engl J Med 2004;351:781-91. [Crossref] [PubMed]
- Cohen SJ, Punt CJ, Iannotti N, et al. Relationship of circulating tumor cells to tumor response, progression-free survival, and overall survival in patients with metastatic colorectal cancer. J Clin Oncol 2008;26:3213-21. [Crossref] [PubMed]
- de Bono JS, Scher HI, Montgomery RB, et al. Circulating tumor cells predict survival benefit from treatment in metastatic castration-resistant prostate cancer. Clin Cancer Res 2008;14:6302-9. [Crossref] [PubMed]
- Allard WJ, Matera J, Miller MC, et al. Tumor cells circulate in the peripheral blood of all major carcinomas but not in healthy subjects or patients with nonmalignant diseases. Clin Cancer Res 2004;10:6897-904. [Crossref] [PubMed]
- Riethdorf S, Fritsche H, Müller V, et al. Detection of circulating tumor cells in peripheral blood of patients with metastatic breast cancer: a validation study of the CellSearch system. Clin Cancer Res 2007;13:920-8. [Crossref] [PubMed]
- De Santis M, Bauernhofer T, Stoiber F, et al. Ciruclating tumor cells (CTC) and HER-2 status on CTC and primary tumor in urothelial cancer (UC) patients refractory to platinum based chemotherapy. J Clin Oncol 2016;34:abstr 4520.
- Michaelson MD, Hu C, Pham HT, et al. A Phase 1/2 Trial of a Combination of Paclitaxel and Trastuzumab With Daily Irradiation or Paclitaxel Alone With Daily Irradiation After Transurethral Surgery for Noncystectomy Candidates With Muscle-Invasive Bladder Cancer (Trial NRG Oncology RTOG 0524). Int J Radiat Oncol Biol Phys 2017;97:995-1001. [Crossref] [PubMed]
- Lobo N, Mount C, Omar K, et al. Landmarks in the treatment of muscle-invasive bladder cancer. Nat Rev Urol 2017;14:565-74. [Crossref] [PubMed]
- Ring A, Smith IE, Dowsett M. Circulating tumour cells in breast cancer. Lancet Oncol 2004;5:79-88. [Crossref] [PubMed]
- Ballestrero A, Garuti A, Bertolotto M, et al. Effect of different cytokines on mammaglobin and maspin gene expression in normal leukocytes: possible relevance to the assays for the detection of micrometastatic breast cancer. Br J Cancer 2005;92:1948-52. [Crossref] [PubMed]
- Xenidis N, Ignatiadis M, Apostolaki S, et al. Cytokeratin-19 mRNA-positive circulating tumor cells after adjuvant chemotherapy in patients with early breast cancer. J Clin Oncol 2009;27:2177-84. [Crossref] [PubMed]
- Danila DC, Anand A, Schultz N, et al. Analytic and clinical validation of a prostate cancer-enhanced messenger RNA detection assay in whole blood as a prognostic biomarker for survival. Eur Urol 2014;65:1191-7. [Crossref] [PubMed]
- Antonarakis ES, Lu C, Wang H, et al. AR-V7 and resistance to enzalutamide and abiraterone in prostate cancer. N Engl J Med 2014;371:1028-38. [Crossref] [PubMed]
- Antonarakis ES, Lu C, Luber B, et al. Clinical Significance of Androgen Receptor Splice Variant-7 mRNA Detection in Circulating Tumor Cells of Men With Metastatic Castration-Resistant Prostate Cancer Treated With First- and Second-Line Abiraterone and Enzalutamide. J Clin Oncol 2017;35:2149-56. [Crossref] [PubMed]
- Katseli A, Maragos H, Nezos A, et al. Multiplex PCR-based detection of circulating tumor cells in lung cancer patients using CK19, PTHrP, and LUNX specific primers. Clin Lung Cancer 2013;14:513-20. [Crossref] [PubMed]
- Wan JC, Massie C, Garcia-Corbacho J, et al. Liquid biopsies come of age: towards implementation of circulating tumour DNA. Nat Rev Cancer 2017;17:223-38. [Crossref] [PubMed]
- Schwarzenbach H, Pantel K. Circulating DNA as biomarker in breast cancer. Breast Cancer Res 2015;17:136. [Crossref] [PubMed]
- Haber DA, Velculescu VE. Blood-based analyses of cancer: circulating tumor cells and circulating tumor DNA. Cancer Discov 2014;4:650-61. [Crossref] [PubMed]
- De Mattos-Arruda L, Caldas C. Cell-free circulating tumour DNA as a liquid biopsy in breast cancer. Mol Oncol 2016;10:464-74. [Crossref] [PubMed]
- Birkenkamp-Demtröder K, Nordentoft I, Christensen E, et al. Genomic Alterations in Liquid Biopsies from Patients with Bladder Cancer. Eur Urol 2016;70:75-82. [Crossref] [PubMed]
- Oellerich M, Schutz E, Beck J, et al. Using circulating cell-free DNA to monitor personalized cancer therapy. Crit Rev Clin Lab Sci 2017;54:205-18. [Crossref] [PubMed]
- Christensen E, Birkenkamp-Demtroder K, Nordentoft I, et al. Liquid Biopsy Analysis of FGFR3 and PIK3CA Hotspot Mutations for Disease Surveillance in Bladder Cancer. Eur Urol 2017;71:961-9. [Crossref] [PubMed]
- Thierry AR, El Messaoudi S, Gahan PB, et al. Origins, structures, and functions of circulating DNA in oncology. Cancer Metastasis Rev 2016;35:347-76. [Crossref] [PubMed]
- Guo W, Gao Y, Li N, et al. Exosomes: New players in cancer Oncol Rep 2017;38:665-75. (Review). [PubMed]
- Soave A, Chun FK, Hillebrand T, et al. Copy number variations of circulating, cell-free DNA in urothelial carcinoma of the bladder patients treated with radical cystectomy: a prospective study. Oncotarget 2017;8:56398-407. [PubMed]
- Luo ZG, Li ZG, Gui SL, et al. Protocadherin-17 promoter methylation in serum-derived DNA is associated with poor prognosis of bladder cancer. J Int Med Res 2014;42:35-41. [Crossref] [PubMed]
- Bettegowda C, Sausen M, Leary RJ, et al. Detection of circulating tumor DNA in early- and late-stage human malignancies. Sci Transl Med 2014;6:224ra24. [Crossref] [PubMed]
- Hauser S, Kogej M, Fechner G, et al. Serum DNA hypermethylation in patients with bladder cancer: results of a prospective multicenter study. Anticancer Res 2013;33:779-84. [PubMed]
- Hauser S, Kogej M, Fechner G, et al. Cell-free serum DNA in patients with bladder cancer: results of a prospective multicenter study. Anticancer Res 2012;32:3119-24. [PubMed]
- Lin YL, Sun G, Liu XQ, et al. Clinical significance of CDH13 promoter methylation in serum samples from patients with bladder transitional cell carcinoma. J Int Med Res 2011;39:179-86. [Crossref] [PubMed]
- Jablonowski Z, Reszka E, Gromadzinska J, et al. Hypermethylation of p16 and DAPK promoter gene regions in patients with non-invasive urinary bladder cancer. Arch Med Sci 2011;7:512-6. [Crossref] [PubMed]
- Ellinger J, El Kassem N, Heukamp LC, et al. Hypermethylation of cell-free serum DNA indicates worse outcome in patients with bladder cancer. J Urol 2008;179:346-52. [Crossref] [PubMed]
- Ellinger J, Bastian PJ, Ellinger N, et al. Apoptotic DNA fragments in serum of patients with muscle invasive bladder cancer: a prognostic entity. Cancer Lett 2008;264:274-80. [Crossref] [PubMed]
- Valenzuela MT, Galisteo R, Zuluaga A, et al. Assessing the use of p16(INK4a) promoter gene methylation in serum for detection of bladder cancer. Eur Urol 2002;42:622-8. [Crossref] [PubMed]
- Dominguez G, Carballido J, Silva J, et al. p14ARF promoter hypermethylation in plasma DNA as an indicator of disease recurrence in bladder cancer patients. Clin Cancer Res 2002;8:980-5. [PubMed]
- Dahse R, Utting M, Werner W, et al. TP53 alterations as a potential diagnostic marker in superficial bladder carcinoma and in patients serum, plasma and urine samples. Int J Oncol 2002;20:107-15. [PubMed]
- Utting M, Werner W, Dahse R, et al. Microsatellite analysis of free tumor DNA in urine, serum, and plasma of patients: a minimally invasive method for the detection of bladder cancer. Clin Cancer Res 2002;8:35-40. [PubMed]
- Utting M, Müller G, Werner W, et al. Detection of tumor genetic alterations of bladder carcinomas in body fluids depends on sample treatment before DNA isolation. Ann N Y Acad Sci 2000;906:67-71. [Crossref] [PubMed]
- D'Arcangelo D, Tinaburri L, Dellambra E. The Role of p16INK4a Pathway in Human Epidermal Stem Cell Self-Renewal, Aging and Cancer. Int J Mol Sci 2017.18. [PubMed]
- Kiselyov A, Bunimovich-Mendrazitsky S, Startsev V. Key signaling pathways in the muscle-invasive bladder carcinoma: Clinical markers for disease modeling and optimized treatment. Int J Cancer 2016;138:2562-9. [Crossref] [PubMed]
- Heidary M, Auer M, Ulz P, et al. The dynamic range of circulating tumor DNA in metastatic breast cancer. Breast Cancer Res 2014;16:421. [Crossref] [PubMed]