Sperm DNA integrity and male infertility: a narrative review and guide for the reproductive physicians
Introduction
Infertility is a highly prevalent condition worldwide, but the exact extent of the phenomenon is difficult to assess, since it has various definitions (1). Current inconsistencies in medical approaches, in addition to individual reproductive choices, allow some cases to go undetected. An epidemiologic study, in which live birth over a 5-year period was measured, reported that the prevalence of couples suffering from infertility was estimated at 48.5 million all over the world (2). According to the definition by the World Health Organization (WHO) (i.e., absence of conception after 12 months of regular, unprotected intercourse), infertility is a condition in which male factors, female factors, or both may contribute, with males being responsible for 50% of the cases overall. A significant percentage of these cases can be attributed to unexplained infertility (UI), where no currently applied knowledge or tools are able to establish a direct diagnosis (3).
Semen analysis (SA) is the backbone of male infertility assessment, however it does not provide any insight into the functional status of spermatozoa and cannot justify adverse reproductive outcomes or UI when the baseline evaluation of both partners is unremarkable (4). The recently published 6th edition of the WHO laboratory manual for the examination and processing of human semen has acknowledged such limitations, and in an attempt to identify and underline the importance of sperm function testing, it has introduced sperm DNA fragmentation (SDF) as an extended examination and recommends this assessment in certain clinical settings, which has been quoted as a major strength of the new manual (5).
SDF occurs in male germ cells when nuclear DNA damage is induced by different factors and is not properly repaired due to dysfunctional repair systems (6). Recent evidence has pointed to the relevance of sperm DNA integrity in reproductive outcomes, since high levels of SDF have been found in infertile men (7) and in association with risk factors for infertility (e.g., drugs, pollutants, lifestyle habits and diseases), which can damage male gametes through testicular apoptosis, altered chromatin maturation and, increased reactive oxygen species (ROS) production (8).
The aim of the present narrative review is to discuss the pathophysiological aspects of SDF including physiological mechanisms that oppose SDF, its causes and significance in terms of reproduction. Additionally, we discuss the different methods of assessment of SDF and provide guidance for potential therapeutic interventions for the management of infertile men with high levels of SDF. Furthermore, we highlight the strengths, weaknesses, opportunities, and threats (SWOT) of current research in SDF and provide insights for future research that may help optimize the diagnostic and predictive potentials of this test in clinical practice. We present the following article in accordance with the Narrative Review reporting checklist (available at https://tau.amegroups.com/article/view/10.21037/tau-22-149/rc).
Methods
To construct this narrative review, a comprehensive literature search was conducted using PubMed from inception with a final endpoint in August, 2021. The search was conducted independently by three authors (AF, GS, and SK) and involved articles pertaining to all aspects of SDF. AF conducted the search for articles on the clinical impact of SDF on male fertility outcomes as well as the treatment options for SDF. Search terms included: “Sperm DNA fragmentation/damage/integrity”, “male infertility”, “assisted reproduction technologies”, “treatment/therapy/management”, and “sperm selection”. GS conducted the search for articles on the molecular aspects of sperm DNA including normal repair and molecular mechanisms of SDF. Search terms included: “Sperm DNA fragmentation/damage/integrity”, “male infertility”, “sperm condensation”, and “sperm DNA repair”. SK conducted the search for articles on the causes and risk factors of SDF as well as the diagnostic tests and guidelines for SDF testing. Search terms included: “Sperm DNA fragmentation/damage/integrity”, “causes/risk factors”, “measurement/diagnosis”, and “guidelines”. All constructed formulas, using the various keywords, were applied to PubMed for a conclusive literature search and included well-designed observational studies and clinical trials, reviews and meta-analyses. Commentaries, editorials, case reports, and non-English articles were excluded. Any uncertainties were resolved by the senior authors AA, RS, and PV. The search strategy is summarized in Table 1.
Table 1
Items | Specification |
---|---|
Date of search | August, 2021 |
Databases | PubMed |
Search terms used | “Sperm DNA fragmentation/damage/integrity”, “male infertility”, “assisted reproduction technologies”, “treatment/therapy/management”, “sperm selection”, “sperm condensation”, “sperm DNA repair”, “causes/risk factors”, “measurement/diagnosis”, and “guidelines” |
Timeframe | Year 2006 to 2021 |
Inclusion and exclusion criteria | Included: observational studies, clinical trials, reviews and meta-analyses |
Excluded: commentaries, editorials, case reports, and non-English articles | |
Selection process | Independent search was conducted by the authors, selecting well-designed observational studies, clinical trials reviews and meta-analyses related to SDF pathogenesis, clinical implication, testing methods, and management |
SDF, sperm DNA fragmentation.
Natural sperm DNA repair
Germ cells can actively react to DNA damage in two ways: apoptosis or DNA repair. Five different mechanisms of sperm DNA repair have been described, which are: nucleotide excision repair (NER), base excision repair (BER), DNA mismatch repair (MMR), post-replication repair (PRR), and double-strand breaks (DSBs) repair. NER acts in the presence of extensive DNA damage (e.g., pyrimidine dimers, bulky adducts, and DNA intra-strand cross-links) and consists of DNA damage detection by XPC/RAD23B proteins, helix unfolding, and cleavage of lesions by XPG and XPF/ERCC1. Conversely, BER operates to remove more limited damage such as non-helix distorting base lesions, which involves recognition and excision of the damaged base through the cleavage of the N-glycosidic bond to deoxyribose by DNA glycosylases. In both pathways, after removal of a single base or surrounding DNA, the gap is then filled by DNA polymerase. MMR, instead, is a security system that verifies the correct replication of DNA by DNA polymerase, playing a key role in maintaining genomic stability (9). On the opposite, PRR mechanisms allow spermatozoa to tolerate single stranded breaks (SSBs), by avoiding fork collapse and accumulation of DSBs, with persistence of DNA damage, but at the cost of reduced DNA replication fidelity (10). Finally, DSB repair mainly occurs via three different pathways: non-homologous end joining, homologous recombination and alternative non-homologous end joining. These mechanisms are significantly different, because homologous recombination uses Rad51 protein and an undamaged homologous template to replace the damaged one, ensuring high fidelity, whereas non-homologous end joining mechanisms act to create compatible ends in DSBs through the removal of non-ligatable termini by the MRE11 complex (9).
Interestingly, in mammals, DSBs repair kinetics in sperm cells differ from any other differentiated cell due to the extremely high grade of chromatin compaction of mature sperm cells that require specific DNA repair proteins. In addition, in cases of DNA damage, the different chromatin compositions between the various spermatogenic cell types influence the recruitment of different sets of repair proteins (11). This partly explains the decline in the ability to repair DNA damage observed in the later stages of spermatogenesis, during which genomic damage can accumulate and be transmitted to the embryo (12). It is worth mentioning as an example, that mature sperm cells are able to initiate the previously described process of BER by 8-oxoguanine DNA glycosylase 1, but they lack the downstream components of this pathway, whereby subsequent DNA repair is strictly oocyte-dependent (13).
An embryo that forms following fusion of a sperm nucleus carrying damaged DNA with an oocyte may exhibit poor developmental prospects, may fail to implant in the uterine lining or may even be aborted at later stages of the first trimester of a clinical pregnancy (14). Normally, the oocyte has its own DNA repair mechanism that are activated following fertilization, which can compensate for the damage in sperm DNA, especially for SSBs involving less than 8% of the sperm genome (15). Therefore, oocyte quality could play an important role in the success of DNA repair. In the process of initial embryo development, the zygote may induce DNA repair for spermatozoon containing SSBs as the complementary DNA strand is present (16-18). However, if the damage is only partially repaired, abnormal development of the embryo may occur (11).
What causes SDF?
Types of SDF
In general, DNA fragmentation is classified into SSBs and DSBs (19,20). In case of SSBs, replication is usually still possible using the other unaffected strand. DSBs are considered to be more harmful than SSBs as they could result in genetic rearrangements. Ribas-Maynou et al. have presented evidence that DSBs are associated with recurrent miscarriage in a couple without a female factor (21). On the other hand, SSBs were reported not to have a significant impact on embryo development or implantation rates (22). Although SSBs and DSBs may have different implications on reproductive outcomes as well as different underlying molecular mechanisms, it is important to point out that most studies investigating the effect of SDF on male infertility and poor reproductive outcomes do not distinguish between the different types of breaks. This may be attributed, at least in part, to the fact that most assays that measure SDF do not distinguish between SSBs and DSBs, as will be discussed later in this review.
Molecular mechanisms of SDF
The active replication of germ cells in the first phase of spermatogenesis can result in transcription errors that could damage the integrity of the inherited genome of the organism. Indeed, DSBs are physiologically induced in spermatocytes during prophase I of meiosis to allow DNA recombination between homologous chromosomes (14). DSBs occurring in meiosis may be potentially dangerous as they can cause chromosomal fragmentation, loss of chromosomal domains, translocations, and other genetic abnormalities (11).
In addition, during the last phases of spermatogenesis, i.e., spermiogenesis, sperm chromatin undergoes DNA condensation, where nearly all the original histones are replaced by protamines, ensuring a high level of compactness in the sperm nucleus (23). Defective chromatin condensation during spermiogenesis which results in an inappropriate protamination and insufficient chromatin packaging, could generate sperm DNA damage (24).
SSBs are caused by DNA ligase activity adjacent to the lesion or by abortive topoisomerase. Topoisomerase is an enzyme which overwinds or unwinds DNA strands. During the process of chromatin compaction in spermiogenesis, topoisomerase II facilitates the creation of breaks in DNA to reduce torsional stress for histone disassembly and chromatin packaging. If these breaks are not modified, consequent impairment of the chromatin packing results in increasing SDF (25,26). DNA ligase is another enzyme that plays a role in joining DNA strands together by catalysing the formation of a phosphodiester bond. Incomplete unwinding or fixation of DNA by abortive function of these enzymes could lead to SSBs.
Furthermore, excessive ROS, such as free radicals and hydrogen peroxide are considered as one of the main causes of SSBs (27,28). They cause DNA base modifications leading to the formation of 8-hydroxy-2’-deoxyguanosine (8-OHdG) and lipid peroxidation. The DNA structure is destabilized by those oxidized base adducts, which results in DNA breaks (29,30). Other bases, including adenosine, cytosine, and thymidine are also sensitive to oxidative damage, although to a lesser extent compared to guanosine (31).
In addition, exogenous sources such as genotoxic drugs and ionizing radiation can directly lead to DNA strand breaks, including DSBs (32). DSBs are also caused as a consequence of SSBs in the process of DNA replication, increased levels of ROS (33), or collapsed replication forks (34).
Risk factors of SDF
Oxidative stress (OS) is caused by an imbalance in homeostasis between ROS production and total antioxidant capacity in semen (35). OS may originate from exogenous sources such as toxicants, smoking, alcohol, diet, and radiation (36-38) or endogenous sources such as varicocele (39). Studies have shown that men with varicocele have significantly higher SDF levels compared to men without varicocele (40,41). A meta-analysis by Wang et al. showed significantly higher levels of SDF in infertile men with varicocele as compared to controls, with an average difference of 9.84% (P<0.00001) (42). Increased scrotal temperature is also known to increase SDF partially through apoptosis induced by OS and elevated expression of specific proteins (43,44). In animal studies, SDF is observed with 2–4 ℃ increase in physiologic scrotal temperature (45,46). Obesity and physical abnormalities such as varicocele or cryptorchidism cause an increase in scrotal temperature and moreover introduce OS. Aging is another factor associated with SDF increase (47,48). This may be attributed to the cumulative exposure to OS and disordered apoptosis that occurs with aging, as well as a decline in antioxidant capacity and a cumulative higher number of spermatogenic cycles in a context where DNA repair activities may decrease and more mutations can be acquired. Genitourinary infections and male genital tract inflammation can also increase ROS production via activation of white blood cells, contributing to SDF (35). Finally, ROS exposure during transfer of spermatozoa from testis to the epididymis after spermatogenesis may also lead to SDF. Longer abstinence could increase SDF as sperm DNA can be damaged by a biological environment of OS while spermatozoa are stored in the epididymis (35). Studies have reported that a shorter abstinence period in ejaculation contributed to decreased ROS levels in semen and sperm DNA fragmentation index (DFI) (49,50). Figure 1 summarizes different etiologies, risk factors, and pathogenesis of SDF.
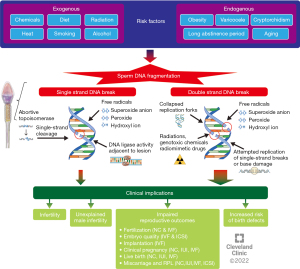
Effect of SDF on fertility outcomes
The growing importance of SDF in the diagnosis and management of male infertility lies in the fact that SDF can have tremendous implications on male fertility and reproductive outcomes. Unexplained male infertility (UMI) refers to infertile men who have no identifiable cause for their infertility and a completely normal SA (51). Sperm from men with UMI has been found to contain significantly higher SDF levels when compared to fertile controls (52,53). In one report, 1 in 4 men with UMI have SDF levels above 20% (53). Idiopathic male infertility (IMI), on the other hand, refers to infertile men who have no identifiable cause for their infertility but do present with abnormalities in their conventional SA (51). Men with idiopathic oligoasthenoteratozoospermia (OAT) have been found to have significantly higher levels of SDF compared to men with normal semen parameters (54). A meta-analysis by Santi et al. has determined that SDF levels of 20% or higher are predictive of male infertility with 79% sensitivity and 86% specificity (7).
Given the large number of studies that have investigated the adverse impact of SDF on reproductive outcomes after natural conception and assisted reproductive technologies (ART), many meta-analyses have been conducted, some of which are summarized in Table 2 (55-68) and are highlighted in the following points:
Table 2
Outcome studied | Method of conception | Number of studies, sample size | Study group vs. comparison | Results | First author, year |
---|---|---|---|---|---|
Fertilization rate | IVF | 4 studies, 770 IVF cycles | High vs. low sperm DNA damage | Lower fertilization rate with high sperm DNA damage (55.4% vs. 71.8%) with (RR =0.79, P=0.23) | Li, 2006 (55) |
ICSI | 3 studies, 201 ICSI cycles | High vs. low sperm DNA damage | Fairly similar fertilization rates for both groups (79.8% vs. 78.2%) with (RR =1.03) | Li, 2006 (55) | |
Embryo quality | IVF or ICSI | 8 studies, 17,879 embryos | High vs. low DFI | Lower rates of good quality embryos with high DFI (42.8% vs. 69.7%) with (RR =0.65, P<0.01) | Deng, 2019 (56) |
Implantation rate | IVF | 8 studies, 4,055 IVF cycles | High vs. low sperm DNA damage | Lower implantation rates after IVF with high sperm DNA damage (RR =0.68, P<0.01) | Ribas-Maynou, 2021 (57) |
ICSI | 11 studies, 3,405 ICSI cycles | High vs. low sperm DNA damage | No difference in implantation rates after ICSI between both groups (RR =0.79, P=0.09) | Ribas-Maynou, 2021 (57) | |
Pregnancy rate | Natural | 3 studies, 616 couples | High vs. low sperm DNA damage | Failure to achieve natural pregnancy with high sperm DNA damage (OR =7.01, P<0.001) | Zini, 2011 (58) |
IUI | 10 studies, 2,839 IUI cycles | High vs. low SDF | Lower pregnancy rates after IUI with high SDF (RR =0.34, P<0.001) | Chen, 2019 (59) | |
3 studies, 917 IUI cycles | High vs. low SDF | Higher pregnancy rates after IUI with lower SDF (RR =3.30, P<0.05) | Sugihara, 2019 (60) | ||
IVF | 11 studies, 1,805 IVF cycles | High vs. low sperm DNA damage | Lower pregnancy rates after IVF with high sperm DNA damage (OR =1.7, P<0.05) | Zini, 2011 (58) | |
5 studies, 816 IVF cycles | High vs. low sperm DNA damage | Lower pregnancy rates with high sperm DNA damage (27.6% vs. 43.1%) with (RR =0.68, P=0.0006) | Li, 2006 (55) | ||
9 studies, 1,268 IVF cycles | High vs. low sperm DNA damage | Lower pregnancy rates after IVF with high sperm DNA damage (OR =0.66, P=0.008) | Zhao, 2014 (61) | ||
16 studies, 3,734 IVF cycles | High vs. low sperm DNA damage | Higher pregnancy rates after IVF with low sperm DNA damage (OR =1.92, P=0.0005) | Simon, 2017 (62) | ||
7 studies, 2,130 IVF cycles | High vs. low DFI | Lower pregnancy rates after IVF with high DFI (RR =0.77, P=0.05) | Deng, 2019 (56) | ||
15 studies, 3,711 IVF cycles | High vs. low sperm DNA damage | Lower pregnancy rates after IVF with high sperm DNA damage (RR =0.72, P=0.02) | Ribas-Maynou, 2021 (57) | ||
ICSI | 14 studies, 1,171 ICSI cycles | High vs. low sperm DNA damage | Pregnancy rates after ICSI are not related to level of sperm DNA damage (OR =1.15, P=0.65) | Zini, 2011 (58) | |
3 studies, 201 ICSI cycles | High vs. low sperm DNA damage | Lower pregnancy rates with high sperm DNA damage (37.7% vs. 49.4%) with (RR =0.76, P=0.09) | Li, 2006 (55) | ||
10 studies, 1,047 ICSI cycles | High vs. low sperm DNA damage | No difference in pregnancy rates after ICSI between both groups (OR =0.94, P=0.65) | Zhao, 2014 (61) | ||
24 studies, 2,282 ICSI cycles | High vs. low sperm DNA damage | Higher pregnancy rates after ICSI with low sperm DNA damage (OR =1.31, P=0.0068) | Simon, 2017 (62) | ||
4 studies, 278 ICSI cycles | High vs. low DFI | No significant difference in pregnancy rates after ICSI between the two groups (RR =0.75, P=0.29) | Deng, 2019 (56) | ||
25 studies, 5,467 ICSI cycles | High vs. low sperm DNA damage | No difference in pregnancy rates after ICSI between both groups (RR =0.89, P=0.09) | Ribas-Maynou, 2021 (57) | ||
Miscarriage rate | IVF | 5 studies, 808 IVF cycles, 345 pregnancies | High vs. low sperm DNA damage | Higher rates of miscarriage with high sperm DNA damage (OR =2.17, P<0.05) | Zini, 2008 (63) |
8 studies, 339 pregnancies | High vs. low sperm DNA damage | Rates of miscarriage after IVF between both groups were not significantly different (OR =1.84, P=0.06) | Zhao, 2014 (61) | ||
7 studies, 1,339 couples | High vs. low DFI | Higher miscarriage rates after IVF with high DFI (RR =1.68, P=0.003) | Deng, 2019 (56) | ||
ICSI | 6 studies, 741 ICSI cycles, 295 pregnancies | High vs. low sperm DNA damage | Higher rates of miscarriage with high sperm DNA damage (OR =2.73, P<0.01) | Zini, 2008 (63) | |
7 studies, 301 pregnancies | High vs. low sperm DNA damage | Higher rates of miscarriage with high sperm DNA damage (OR =2.68, P=0.003) | Zhao, 2014 (61) | ||
4 studies, 96 couples | High vs. low DFI | Higher miscarriage rates after ICSI with high DFI (RR =3.38, P=0.005) | Deng, 2019 (56) | ||
Variable | 16 studies, 1,252 pregnancies | High vs. low sperm DNA damage | Significantly higher rates of miscarriage with high sperm DNA damage (RR =2.16, P<0.00001) | Robinson, 2012 (64) | |
Delivery rate/live birth rate | IUI | 2 studies, 518 IUI cycles | High vs. low DFI | Lower delivery rates after IUI with high SDF (RR =0.14, P<0.001) | Chen, 2019 (59) |
IVF | 4 studies, 553 couples | High vs. low SDF | Higher live birth rates after IVF with low SDF (RR =1.27, P=0.01) | Osman, 2015 (65) | |
6 studies, 1,634 IVF cycles | High vs. low sperm DNA damage | Lower live birth rates after IVF with high sperm DNA damage but non-significant (RR =0.48, P=0.06) | Ribas-Maynou, 2021 (57) | ||
ICSI | 5 studies, 445 couples | High vs. low SDF | Higher live birth rates after ICSI with low SDF (RR =1.11, P=0.04) | Osman, 2015 (65) | |
9 studies, 3,017 ICSI cycles | High vs. low sperm DNA damage | No relationship between sperm DNA damage and live birth rate after ICSI (RR =0.92, P=0.62) | Ribas-Maynou, 2021 (57) | ||
IVF or ICSI | 10 studies, 1,785 IVF or ICSI cycles | High vs. low DFI | No difference in live birth rate between both groups (61.9% vs. 62.3%) with (RR =0.89, P>0.05) | Deng, 2019 (56) | |
Recurrent pregnancy loss | Not specified | 13 studies, 517 cases and 384 controls | Couples with RPL vs. Fertile couples | Higher rates of SDF among couples with RPL with a mean difference of 10.7%, P<0.0001 | McQueen, 2019 (66) |
Natural | 13 studies | Couples with RPL vs. Fertile couples | Higher rates of SDF among couples with RPL with a mean difference of 11.98%, P<0.001 | Tan, 2018 (67) yet the relationship between DNA fragmentation in the male gamete and idiopathic recurrent pregnancy loss (RPL | |
Not specified | 18 studies, 1,181 cases and 925 controls | Couples with RPL vs. Fertile couples | Higher DFI among couples with RPL with a standard mean difference of 1.6, P<0.00001 | Li, 2021 (68) |
SDF, sperm DNA fragmentation; DFI, DNA fragmentation index; IUI, intrauterine insemination; IVF, in vitro fertilization; ICSI, intracytoplasmic sperm injection; RPL, recurrent pregnancy loss; RR, relative risk; OR, odds ratio.
- SDF can reduce the ability of a couple to conceive naturally.
- SDF can reduce pregnancy rates and delivery rates after intrauterine insemination (IUI).
- SDF can reduce fertilization rates, implantation rates, pregnancy rates, and impair embryo quality after in vitro fertilization (IVF), as well as lead to higher rates of miscarriage and lower live birth rates.
- SDF has no effect on fertilization rates or pregnancy rates after intracytoplasmic sperm injection (ICSI), but it does impair embryo quality and is associated with a higher risk of miscarriage and may lead to lower live birth rates.
- Elevated SDF can lead to recurrent pregnancy loss (RPL).
When looking at pregnancy rates with different methods of conception, it is clear that elevated SDF impairs pregnancy after natural conception, IUI, and IVF. Regarding ICSI, although the meta-analysis by Simon et al. have shown reduced pregnancy with high SDF (62), most other analyses have demonstrated no associations or small non-significant associations between the level of SDF and pregnancy rates after ICSI (56-58,61). Regarding fertilization rates, Li et al. showed lower fertilization rates after IVF with high SDF, but no difference in rates of fertilization with ICSI (55). A retrospective study demonstrated a significant negative correlation between DFI and fertilization rate after IVF (69). The most recent meta-analysis by Ribas-Maynou et al. reported data on implantation rates being affected with elevated SDF after IVF but not significantly after ICSI (57). Conversely, it is important to point out that not all studies have reported an association between SDF and reproductive outcomes after IVF or ICSI (70).
Differences in the IVF and ICSI procedures can explain the differential effect of SDF on fertilization and pregnancy rates between the two procedures. In IVF, spermatozoa are co-incubated with the oocytes and fertilization is allowed to proceed randomly as one spermatozoon penetrates the zona of the oocyte. This also allows spermatozoa to be exposed to OS from the surrounding environment as well as the supporting cumulus and corona cells of the oocyte during culture, adding to the SDF and impairing fertilization and ability to achieve pregnancy (71,72). Furthermore, SDF level was inversely correlated to normal motility and morphology of sperm cells when used in IVF (73). In ICSI, on the other hand, the sperm cell is manually selected through micromanipulation and based on morphological and kinetic criteria and directly injected into the oocyte after all surrounding cells are removed, bypassing the random fertilization process that occurs with IVF and minimizing exposure to OS.
During the early divisions of embryonic development, fragmented male genome is unequally distributed between daughter cells, leading to a phenomenon called chaotic mosaicism; meaning cells of the developing embryo do not contain an identical and balanced genotype. This has been attributed as a major cause of miscarriage resulting in a reduced live birth rate following ART, as well as repeated miscarriage incidents with RPL (74). If the pregnancy progresses to live birth, damaged sperm leading to chromosomal abnormalities may lead to congenital anomalies in the offspring (74).
Data on the effect of SDF on live birth rate is more heterogeneous. The recent meta-analysis by Ribas-Maynou et al. reported non-significant association between sperm DNA damage and live birth rate after IVF and no associations between the two after ICSI (57). This is in line with the meta-analysis by Deng et al. that reported no association (56), but in conflict to the results of a previous analysis by Osman et al. that reported significantly reduced live birth rates with high SDF after both IVF and ICSI (65).
The majority of the meta-analyses presented in this review and in Table 2, have indicated as a limitation, the variability of the different observational studies, investigating the effects of elevated SDF on reproductive outcomes, that were included and analysed. This weakens the conclusions set forth by these meta-analyses. Therefore, well-designed studies that account for different patient factors and control for heterogeneity are needed to reach more solid conclusions on the effect of SDF on the various reproductive outcomes.
How to select a test for sperm DNA fragmentation?
Implementing SDF into clinical practice: Guidelines of Professional Societies and the WHO 6th Edition
Currently, the American Society for Reproductive Medicine (ASRM) guidelines do not recommend routine SDF test in evaluation of male infertility due to lack of information (75-77). On the other hand, the European Society of Human Reproduction and Embryology (ESHRE) guidelines states that SDF testing could be considered for explanatory purposes in couples with RPL (78). Similarly, the European Association of Urology (EAU) guidelines in 2020 also recommend SDF test in couples with RPL following natural pregnancy and male partners with UI (79).
In the latest WHO Manual, SDF is recognized as an important indication in the practice of male infertility, becoming one of the most discussed and promising biomarkers in andrology (80). The WHO also picked up supportive evidence between SDF and embryo development, implantation, spontaneous pregnancies and pregnancies in assisted reproduction (62,64,67,81). In the section of extended testing, the WHO manual provides the detailed procedures of the four commonly used assays for determining the percentage of SDF in any given semen sample; Terminal deoxynucleotidyl transferase deoxyuridine triphosphate (dUTP) nick end labelling (TUNEL), acridine orange flow cytometry (AO-FCM) also known as sperm chromatin structure assay (SCSA), sperm chromatin dispersion test (SCD), and single cell gel electrophoresis assay (Comet assay) (82). The manual also states that the diagnostic thresholds of these assays should be determined and validated by the performing laboratory and according to the procedure utilized.
Summary of common tests for assessment of SDF
Currently there is no globally accepted guideline regarding the choice of assays for SDF testing. The choice of assay in clinical practice depends on trained personnel, availability for instrumentation including cost, and running-time in each clinic. Major practical points for each test are reviewed in Table 3.
Table 3
Assay | Direct or indirect | Principle | Type of DNA fragmentation | Advantages | Disadvantages |
---|---|---|---|---|---|
TUNEL | Direct | Labelling of free 3’-OH nicks with dUTP at the DNA break | SSBs & DSBs simultaneously | • Highly standardized protocol | • More technically demanding–equipment training |
• Minimal inter-observer variability | • No clear cut-off values | ||||
• Testing can be done on both fresh and frozen specimens | |||||
SCSA | Indirect | Evaluation of DNA integrity with acid denaturation followed by Acridine Orange staining | SSBs & DSBs simultaneously | • Simultaneous examination of a large number of cells | • More technically demanding–equipment training |
• Highly standardized protocol | • Commercial kits are not available | ||||
• Testing can be done on both fresh and frozen specimens | |||||
SCD | Indirect | Evaluation of the dispersed chromatin (“halo”) after lysis of sperm membranes | SSBs & DSBs simultaneously | • Highly standardized protocol | • Inter-observer variability |
• No special equipment training required | |||||
• Both fresh and frozen-thawed samples can be used | |||||
Comet | Direct | Single-cell gel electrophoretic separation | SSBs and/or DSBs | • Possibility to detect SSBs and DSBs separately at the same time | • Poor repeatability |
• Both fresh and frozen-thawed samples can be used | • High inter-observer variability | ||||
• Non standardized protocols and thresholds |
SDF, sperm DNA fragmentation; TUNEL, terminal deoxynucleotidyl transferase dUTP nick end labelling; SCSA, sperm chromatin structure assay; SCD, sperm chromatin dispersion; SSBs, single-stranded breaks; DSBs, double-stranded breaks.
TUNEL
TUNEL is currently the most commonly used SDF assay worldwide. It is based on technique to label free 3’-OH nicks with dUTP at the DNA break (83). The 3’-OH-termini of the DNA strand breaks work as primers and become labelled in this procedure with bromodeoxyuridine (Brd-U) in a reaction catalysed by Terminal deoxynucleotidyl Transferase (TDT), which is a template-independent DNA polymerase (84). The method can directly assess both SSBs and DSBs. d-UTP is the substrate that is added by the TDT enzyme to the free 3’-OH-termini. The added d-UTP can be labelled directly and plays a role as a direct marker of breaks in DNA, or its signal can be amplified by using a modified d-UTP to which labelled anti-d-UTP antibody can be adsorbed (85). Although this method needs flow cytometry, TUNEL is able to analyse up to 2.5×106 spermatozoa, which is a higher number of cells compared to other methods (86). A DFI threshold for infertile men as measured by TUNEL was found to be 17% in one report, with a specificity of 91.6% and a sensitivity of 32.6% (87). As a limitation, TUNEL cannot detect DNA fragmentation produced by nucleases generating 3’-PO4/5’-OH breaks, because the TdT enzyme used in TUNEL does not react with 3’-PO4 ends and the T4 DNA ligase in the ligation assay is unable to attach the probe to 5’-OH ends in DNA (88).
SCSA
The method of SCSA is based on the action of AO. It emits green fluorescence when it is introduced between double-stranded DNA, and it also emits red fluorescence if it is related to single-stranded DNA (82). This method can be used to assess fresh or frozen samples and comprises only two main methodological steps. In the first step, spermatozoa are treated with a low pH (1.2) detergent solution to denature their chromatin and allow its subsequent staining. In the second step, the sperm suspension is neutralized, and the cells are stained with a second solution containing AO. Data for red and green fluorescence obtained from analyzing 5,000–10,000 spermatozoa per sample are collected by flow cytometry and transformed. The degree of red fluorescence in the sperm population corresponds to DFI, which represents the percentage of cells with broken DNA. The analysis of the collected data can be done in any flow cytometric software. SCSA also needs flow cytometry, similar to TUNEL assay, and is also able to analyse many (5,000–10,000) spermatozoa within few seconds and thus provides a less subjective result (89). Moreover, this method also reports % high DNA stainability (HDS) which indicates the extent of stainability of the sperm nucleus, representing immature spermatozoa (90). The DFI threshold for male infertility diagnosis using SCSA is reported as 20–25% (7). In a prospective study on 1,316 infertile couples who underwent IVF and 266 who underwent ICSI, a DFI threshold using SCSA of <11.3% was predictive of pregnancy success after IVF with 56.1% sensitivity and 60% specificity, and a threshold of <30.3% was predictive of pregnancy success after ICSI with 50.6% sensitivity and 68.8% specificity (91). In this study, thresholds were determined using ROC analysis.
SCD
SCD test is a method to evaluate the sensitivity of sperm DNA to acid denaturation. The principle of SCD is based on the fact that intact DNA loops expand following denaturation and extraction of proteins in the nucleus, whereas when DNA is fragmented, dispersion does not develop or is minimal. This method relies on the capacity of the intact sperm chromatin to create dispersion halos after exposure to acid and a lysing solution; the halos correspond to loosened DNA loops attached to the residual structure of nuclear, which are released after the removal of nuclear proteins. The DNA breaks prevent this dispersion as they are susceptible to denaturation (92). Different from TUNEL and SCSA, this method does not need any advanced equipment and can be performed in a private clinic or office setting using commercially available assay kits. However, SCD analyses only 300–500 spermatozoa manually under the microscope, which can create inter-observer variability (20). DFI thresholds to diagnose male infertility using SCD range from 16–30%, but 20% is considered to be the best (7). In one report, a threshold of 22.5% SDF using SCD, with 76.8% sensitivity and 92.9% specificity was predictive of achieving pregnancy after natural conception (21).
Comet assay
The name of the test is related to the ‘comet’ appearance under fluorescence microscope of the stained unwound DNA fragments that are detached from the sperm head after electrophoretic movement. It is a method to evaluate SDF in individual sperm based on the differential migration of broken DNA strands influenced by an electric field depending on the size and charge of the strands (19). In Comet, the spermatozoa are migrated in an agarose matrix, wherein a detergent-induced lysis is promoted under high salt conditions. During an electrophoresis step, the broken DNA strands migrate towards the anode, generating the characteristic dispersion pattern that resembles a comet tail. The intact DNA constitutes the comet’s head, while the fragmented strains of DNA constitute the comet’s tail. The relative fluorescence of this last element compared to the head serves as a measure of the amount of DNA damage. The average amount of DNA fragmentation across is shown as the average Comet score, which is a calculated by the analysis of 100 individual comets (spermatozoa) (93). Neutral Comet assay uses only controlled protein depletion to detect DSBs selectively while the 2-dimensional Comet assay applies two electrophoretic runs to map SSBs and DSBs simultaneously (94). Alkaline Comet Assay, where a buffer of pH >13 is used, can detect SSB more sensitively compared to neutral (95), and it is now more commonly used in andrology labs than the neutral assay. In one report, a threshold of 26% by alkaline comet (average comet score) is reported to discriminate fertile from infertile men with an AUC of 0.925 (sensitivity: 73%; specificity: 100%) (96). Similar to SCD, Comet assay does not need advanced equipment but only commercially available assay kits. As descried above, among the four currently available methods, Comet has the ability to distinguish between SSBs and DSBs. However, only a small number [100] of spermatozoa can be analysed manually, the same as SCD, which creates a chance for inter-observer variability (20).
SDF testing thresholds
SDF thresholds to enable precise diagnosis and management are lacking. The cut-off values reported in the literature demonstrate an increased variability, ranging between the extremes of 4% to 56% and this uncertainty persisted over the last 20 years despite significant data contribution from several research groups (20). This situation has been proven difficult to resolve due to multiple factors surrounding SDF testing such as; the variability of the techniques, the study population, the infertility factor, the reproductive outcomes tested and the laboratory specialization and experience. Additionally, potential intrinsic and extrinsic factors that may impact the interpretation of SDF test results such as; female factor infertility, patient’s age, basic and advanced semen variables, genetic repair mechanisms, semen processing and conditions, ART method are not fully addressed. Furthermore, the standardization of SDF assessment was not resolved in the latest 6th Edition of the WHO manual for human SA (5). Although the manual highlights the indications of SDF testing and provides an updated guide on laboratory protocols of the few techniques, it lacks threshold definition for each test and recommends adopting individual reference ranges according to each laboratory operational procedures and clinical experience on representative values, echoing fertility dynamics.
In light of this heterogeneity in SDF testing methods and thresholds, a recent meta-analysis by Santi et al., suggested a universal cut-off value of 20% for the discrimination between fertile and infertile males (7). A recent study by Agarwal et al. also suggested a unanimous 20% cut-off for all laboratory assessment methods (20). Similarly, Esteves et al. suggested a 20% threshold for SCSA, TUNEL, and SCD, but differed in the assessment through Comet method by suggesting a cut-off value of 26% (97). In terms of reproductive outcome prediction, Esteves et al., concluded that SDF values of 20–30% appear to correlate with adverse pregnancy outcomes through either natural conception or assisted reproduction (97). Nevertheless, formal guidelines are warranted to alleviate the existing discrepancies and that can be clinically implemented to utilize the crucial information offered through this diagnostic utility (98).
Management of elevated SDF
Several strategies can be employed to reduce SDF among infertile men before natural or assisted reproduction to improve reproductive outcomes. Known underlying causes and risk factors of high SDF such as smoking and environmental exposures should be addressed (98). Also, suitable antibiotic therapy should be considered for male genital tract infections which are associated with elevated SDF (99). The different strategies that can be used to improve sperm DNA integrity are summarized in Figure 2 and are discussed in the subsequent sections.
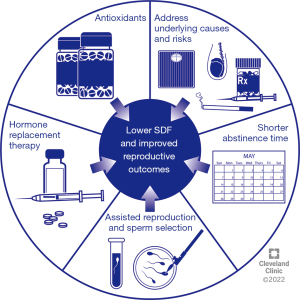
Weight loss
SDF was found to be significantly higher among obese men [body mass index (BMI) ≥30 kg/m2] and men with metabolic syndrome (100-102). Therefore, weight loss as a means to reduce SDF, has been studied. In a prospective study, 105 infertile men with above than normal BMI were given a diet and exercise weight loss program for 12 weeks, and reported significant reductions in their mean SDF after they completed the program (103). The impact of surgical weight loss on SDF has also been studied with promising results (104,105).
Varicocele repair
Men with clinical varicocele were found to have significantly elevated SDF levels compared to healthy controls (106). Varicocelectomy can reduce SDF levels and improve fertility outcomes in this population of infertile men. A prospective study that included 60 infertile men with clinical varicocele reported significant reductions in their mean SDF from 29.5% to 18.8% (P<0.001), 3–6 months after varicocelectomy (107). A recent meta-analysis including 394 infertile men with clinical varicocele indicated significant reduction of SDF following varicocele repair (108). Furthermore, significantly improved clinical pregnancy and live birth rates after ICSI were reported in the group of infertile men with clinical varicocele that underwent repair (109).
Antioxidants
Given the important role of seminal OS in the pathogenesis of SDF (8), many researchers have investigated the use of different antioxidants to improve sperm DNA integrity and fertility outcomes. For example, Jannatifar et al. supplied 50 infertile men with n-acetyl cysteine for 3 months and reported a significant decline in their mean SDF from 19.3% to 15.1% (P=0.01) (110). Alahmar et al. studied the impact of co-enzyme Q10 supplementation to 65 infertile men with idiopathic oligoasthenozoospermia, and also reported significant reductions in SDF from 35.6% to 30.9% (P<0.001) after treatment, as well as improvement in conventional semen parameters and markers of OS (111). In 2005, Greco et al. treated infertile men who have failed an initial cycle of ICSI and had ≥15% SDF with a combination of daily vitamin C and vitamin E, and reported significantly improved pregnancy and implantation rates with the second ICSI attempt after two months of treatment (112).
On the other hand, some studies have published contradicting results. The MOXI trial was a multi-center randomized clinical trial that included 171 infertile men with at least one abnormal conventional semen parameter or SDF ≥25%, who received either a combination antioxidant regimen for 3–6 months or a placebo. After 3 months of treatment, there was no significant difference in percentage DNA fragmentation between the antioxidant and the placebo group. Furthermore, reproductive outcomes including clinical pregnancy rates, first trimester miscarriage rates and live birth rates were fairly similar between both groups (113). Another randomized clinical trial supplied infertile men with a combination of zinc and folic acid or a placebo for 6 months, and reported significantly higher DFI among the group that received antioxidants with a statistically significant mean difference of 2.4% (114). To explain this adverse outcome of antioxidants on SDF, it is important to keep in mind that not all SDF is caused by OS, with other pathophysiologic mechanisms playing a role as discussed earlier in this review. In fact, any disruption of the redox balance within the male reproductive system may lead to infertility, and supplying excessive or unwarranted antioxidants may tilt the scale towards reductive stress which, similar to OS, can also cause infertility by affecting the physiologic functions of endogenous ROS such as sperm capacitation and sperm DNA integrity (115). Supplying antioxidants may impair adequate maturation of the sperm nucleus, as excessive antioxidants prevent the creation of disulfide bridges between protamines, making sperm DNA less condensed and more susceptible to exogenous insults and damage, therefore increasing SDF (31).
Overall, several studies that explore the effects of antioxidants therapy on SDF have been published (116,117). Various antioxidant regimens, whether single agents or combination therapy, were supplied to the populations of infertile men by the different studies and for different treatment durations, mostly ranging from two to six months. The population of infertile men included also differed among the different studies, for example some included infertile men with abnormal conventional semen parameters, others have also looked into seminal OS, while others have included infertile men of couples who have failed ART or infertile men with known underlying conditions such as subclinical varicocele, leukocytospermia, and environmental exposure and studied the impact of antioxidant supplementation in them. The heterogeneity of the various studies in terms of patient selection, antioxidant regimen and dose, duration of treatment, and different conditions that may be associated with SDF, together with the scarcity of well-designed randomized clinical trials and the contradicting evidence published, as well as the potential harms have precluded the ability to make specific recommendations on the appropriate antioxidant treatment regimen to infertile men in general, and in those with elevated SDF in particular.
Shorter abstinence
A shorter ejaculatory abstinence is associated with lower levels of SDF, with semen collected after 1–2 days of abstinence containing significantly less SDF (9–10%) as measured by TUNEL assay, and semen collected after 9–11 days of abstinence containing significantly higher SDF (15–21%) (P<0.001) (118). This has also been demonstrated on clinical grounds with improved reproductive outcomes after IUI with an improved clinical pregnancy rate when an ejaculatory abstinence of two or less days was used compared to three or more days (11.3% vs. 6–7.3%; P<0.05) (119) and significantly improved pregnancy rates after ICSI, when recurrent ejaculation was performed in the last four days before the procedure and a final abstinence period of 12 hours was used (120). Therefore, reduced ejaculatory abstinence and recurrent ejaculation can be recommended to men with elevated SDF in an attempt to improve ART outcomes (98).
Hormonal therapy
Follicle stimulating hormone (FSH) is a commonly prescribed medical therapy for men with IMI and has been associated with improved semen parameters and fertility outcomes (121). FSH has also been shown to improve DNA integrity, as demonstrated by a meta-analysis that included 6 studies and reported a small but significant improvement in SDF after FSH therapy (7). However, the evidence presented by this analysis is not reliable, as the included studies are very heterogeneous in terms of the patients included, the regimen of hormonal therapy, and the methods of measuring SDF (122). Furthermore, a pharmacogenetic study found that FSH treatment significantly improves SDF only in men with IMI that show NN genotype of the FSH receptor, but not the SS genotype (123). Given the poor quality of evidence and the possible genetic contribution to response, FSH therapy was not included in the recently published guidelines regarding the approach and management of SDF (20,97).
Advanced sperm selection
Given the less impact of SDF on pregnancy rates after ICSI, couples with persistently elevated SDF may be directed to this ART procedure (20). However, elevated SDF may still be associated with miscarriage, even after ICSI. Several methods of advanced sperm selection can be used to reduce SDF before sperm is used for any ART procedure. These include:
- Magnetic activated cell sorting (MACS): uses Annexin V beads to bind phosphatidylserine on the surface of apoptotic sperm and removes them, thereby lowering SDF (124). In a retrospective study, the outcomes of ICSI performed for infertile men with SDF >20% as measured with TUNEL, were compared between 366 men for whom MASC was performed after density-gradient centrifugation (DGC) and 358 men who underwent ICSI after DCG without MACS (125). Although fertilization rates were similar for both groups, the MASC group had significantly higher clinical pregnancy (60.7% vs. 51.5%; P=0.014), and live birth rates (47.4% vs. 31.2%; P=0.001), and lower miscarriage rate (14.7% vs. 20.6%; P=0.034) compared to the DCG only group.
- Intracytoplasmic morphologically selected sperm injection (IMSI): uses microscopy to choose motile sperm devoid of vacuoles in their head, which have been found to contain less SDF (126). In a trial that included 80 infertile men, SDF measured by TUNEL was significantly lower in the IMSI group compared to the ICSI group (P<0.0001) (127). A meta-analysis that included studies comparing IMSI and ICSI outcomes for infertile men, reported significantly improved implantation rates (OR =1.56; P=0.01) and pregnancy rates (OR =1.61; P=0.004) for IMSI compared to ICSI, but no difference in miscarriage rates (128).
- Physiologic ICSI (P-ICSI): uses hyaluronic acid binding to choose mature sperm which were found to contain significantly lower SDF compared to initial unprocessed sperm (5.3% vs. 16.5%; P<0.001) (129). When compared to MACS, no significant differences were found for implantation, clinical pregnancy, and ongoing pregnancy rates overall, but when stratified for female age, outcomes were better when P-ICSI was used for women older than 30 versus MACS, although not statistically significant (130).
- Microfluidic sperm sorters: passes sperm through microchannels using fluid dynamics to choose motile sperm, which have been found to have significantly lower DFI compared to sperm processed by conventional techniques of centrifugation and swim-up (0.8% vs. 10.1%; P=0.0014) (131).
- Testicular-derived sperm: a meta-analysis that included studies comparing SDF and reproductive outcomes between testicular sperm and ejaculated sperm concluded that SDF is significantly lower in testicular sperm (8.9% vs. 33.4%, P<0.0001) and when used for ICSI, is associated with significantly improved reproductive outcomes including clinical pregnancy (OR =2.42, P<0.001) and live birth rates (OR =2.58, P<0.001) and lower miscarriage rates (OR =0.28, P=0.005) (132).
When discussing sperm selection, it is important to point out that little evidence is available regarding the benefit in improving SDF and reproductive outcomes. Furthermore, conflicting evidence exists, for example in a retrospective study on IMSI, sperm head vacuoles were not associated with SDF or live birth rates (133). Well-designed clinical trials that investigate the effect of the various sperm selection methods for infertile men and the impact on ART outcomes are needed in order to make strong recommendations. As for testicular sperm, although it has been recommended for use as a last resort in cases of elevated SDF by the recent guidelines, the evidence behind the use of this invasive method is of poor quality and with no valid SDF testing for testicular sperm (98). Furthermore, a prospective study investigating the outcomes of ICSI with testicular sperm compared to ejaculated sperm, in infertile men with elevated SDF and previous ICSI failure, reported no benefit of testicular sperm in improving reproductive outcomes (134).
Future of SDF research: a SWOT analysis
Recently, two different guidelines on SDF, based on high-quality evidence, have been published by two research groups (20,97). In order to evaluate the current state of research on SDF, we analysed these sources by applying the SWOT (strengths, weaknesses, opportunities, and threats) analysis framework, a model derived from the business world that has already been used in the field of reproductive medicine research (135). The results are presented in Figure 3.
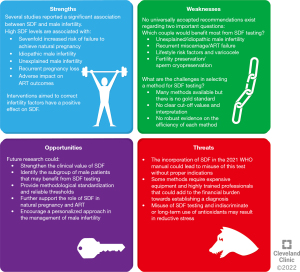
Strengths
Several studies reported that SDF negatively impacts male fertility. In particular, SDF levels inversely correlate with probability of natural pregnancy and high SDF levels are associated with a 7-fold increased risk of failure to achieve natural pregnancy (20). Moreover, high SDF can be found in up to 20% (97) or 30% (20) of idiopathic infertile couples and in subjects with varicocele (20). Higher levels of SDF were also observed in couples with RPL than in fertile couples (20,97). Interventions aimed to correct infertility factors such as varicocelectomy and changes in lifestyle habits have a positive effect on OS and SDF (20,97).
Weaknesses
Unfortunately, despite the validity of SDF as an infertility marker, there are uncertainties about its actual clinical application. In particular, two important questions remain unanswered: which couples would benefit most from SDF testing and how should the test be conducted? To answer the first question, Agarwal et al. suggested SDF testing in men with UMI, IMI, recurrent miscarriage, lifestyle risk factors, varicocele and recurrent ART failure (Grade C recommendation—low quality of evidence). Esteves et al. offer similar indications (recommendation grade based on levels of evidence B-D—moderate-very low quality of evidence) and suggest SDF testing also for men undergoing sperm cryopreservation (Grade D recommendation). Regarding the best investigation technique and optimal cut-offs, Agarwal et al. conclude that the TUNEL assay is the most commonly used and it is reliable and accurate, but high heterogeneity of published data exists to establish clear cut-off values. Esteves et al. provide a review of all the limitations and advantages of the different methods, but do not express a preference for any of them (20,97).
Opportunities
Given that the association between SDF and male infertility is strong, future efforts should focus on investigating the possible practical uses of this parameter. In particular, it is necessary to establish which subgroup of infertile couples can benefit most from the identification of high levels of SDF. Also, standardisation of the testing methods and providing reliable references values are warranted. Since reducing SDF levels could be a valid objective in the treatment of infertility, it could be an excellent opportunity for high-quality interventional research.
Threats
Despite the introduction of SDF assay in the 6th edition of WHO laboratory manual as an important extended test of semen (136), there are still many uncertainties about this type of investigation. Its indiscriminate use may not bring benefits outside specific contexts that should be identified. Furthermore, some tests such as the TUNEL assay require expensive equipment and need highly trained laboratory personnel.
Conclusions: summary of key points
- Currently, SDF is recognized as an important parameter in the practice of male infertility. There is growing evidence that high SDF negatively impacts reproductive outcomes under natural and ART conditions.
- Defects in sperm chromatin packaging, apoptosis, and excessive seminal OS are underlying pathophysiological mechanisms that lead to SDF, which is associated with many conditions and risk factors including: aging, varicocele, genital tract infections, poor lifestyle habits, and noxious environmental or occupational exposure.
- The procedures that are commonly used for SDF assessment include the TUNEL, SCSA, SCD, and Comet. The choice of assay depends on trained personnel and availability of instrumentation, and the diagnostic thresholds should be determined and validated by the performing laboratory.
- The ASRM guidelines do not recommend routine SDF test in the evaluation of male infertility due to lack of information. On the other hand, the ESHRE and EAU guidelines consider SDF in couples with RPL. The WHO 2021 manual describes SDF testing as a potentially important addition in the work-up of infertile men.
- Several strategies are suggested to reduce SDF among infertile men before natural or assisted conception including: (I) control of body weight; (II) reduce smoking and environmental exposures; (III) treatment of genital tract infection; (IV) varicocele repair; and (V) antioxidant therapy. In addition, several methods of sperm selection can be used in ART procedure including MACS, IMSI, P-ICSI or testicular sperm.
- Strong evidence is available on the negative impact of SDF on male fertility potential. However, the quality of evidence and the heterogeneity of the available studies prevent the ability to make strong recommendations regarding indications of SDF testing, assays for SDF testing and their cut-off values, and treatment of infertile men with elevated SDF.
Acknowledgments
The authors would like to thank Dr. Damayanthi Durairajanayagam for her critical review and editing of this manuscript and for her advice on the figures. The authors would like to thank Professors Ralf Henkel and Florence Boitrelle for their critical review and advice on the figures. Authors are thankful to the artists from the Cleveland Clinic’s Center for Medical Art & Photography for their help with the design of the figures.
Funding: None.
Footnote
Reporting Checklist: The authors have completed the Narrative Review reporting checklist. Available at https://tau.amegroups.com/article/view/10.21037/tau-22-149/rc
Conflicts of Interest: All authors have completed the ICMJE uniform disclosure form (available at https://tau.amegroups.com/article/view/10.21037/tau-22-149/coif). The authors have no conflicts of interest to declare.
Ethical Statement: The authors are accountable for all aspects of the work in ensuring that questions related to the accuracy or integrity of any part of the work are appropriately investigated and resolved.
Open Access Statement: This is an Open Access article distributed in accordance with the Creative Commons Attribution-NonCommercial-NoDerivs 4.0 International License (CC BY-NC-ND 4.0), which permits the non-commercial replication and distribution of the article with the strict proviso that no changes or edits are made and the original work is properly cited (including links to both the formal publication through the relevant DOI and the license). See: https://creativecommons.org/licenses/by-nc-nd/4.0/.
References
- Inhorn MC, Patrizio P. Infertility around the globe: new thinking on gender, reproductive technologies and global movements in the 21st century. Hum Reprod Update 2015;21:411-26. [Crossref] [PubMed]
- Mascarenhas MN, Flaxman SR, Boerma T, et al. National, regional, and global trends in infertility prevalence since 1990: a systematic analysis of 277 health surveys. PLoS Med 2012;9:e1001356. [Crossref] [PubMed]
- Vander Borght M, Wyns C. Fertility and infertility: Definition and epidemiology. Clin Biochem 2018;62:2-10. [Crossref] [PubMed]
- Wang C, Swerdloff RS. Limitations of semen analysis as a test of male fertility and anticipated needs from newer tests. Fertil Steril 2014;102:1502-7. [Crossref] [PubMed]
- Boitrelle F, Shah R, Saleh R, et al. The Sixth Edition of the WHO Manual for Human Semen Analysis: A Critical Review and SWOT Analysis. Life (Basel) 2021;11:1368.
- Agarwal A, Panner Selvam MK, Baskaran S, et al. Sperm DNA damage and its impact on male reproductive health: a critical review for clinicians, reproductive professionals and researchers. Expert Rev Mol Diagn 2019;19:443-57. [Crossref] [PubMed]
- Santi D, Spaggiari G, Simoni M. Sperm DNA fragmentation index as a promising predictive tool for male infertility diagnosis and treatment management - meta-analyses. Reprod Biomed Online 2018;37:315-26. [Crossref] [PubMed]
- Muratori M, Marchiani S, Tamburrino L, et al. Sperm DNA Fragmentation: Mechanisms of Origin. Adv Exp Med Biol 2019;1166:75-85. [Crossref] [PubMed]
- Gunes S, Al-Sadaan M, Agarwal A. Spermatogenesis, DNA damage and DNA repair mechanisms in male infertility. Reprod Biomed Online 2015;31:309-19. [Crossref] [PubMed]
- Gao Y, Mutter-Rottmayer E, Zlatanou A, et al. Mechanisms of Post-Replication DNA Repair. Genes (Basel) 2017;8:64. [Crossref] [PubMed]
- González-Marín C, Gosálvez J, Roy R. Types, causes, detection and repair of DNA fragmentation in animal and human sperm cells. Int J Mol Sci 2012;13:14026-52. [Crossref] [PubMed]
- Marchetti F, Wyrobek AJ. DNA repair decline during mouse spermiogenesis results in the accumulation of heritable DNA damage. DNA Repair (Amst) 2008;7:572-81. [Crossref] [PubMed]
- Smith TB, Dun MD, Smith ND, et al. The presence of a truncated base excision repair pathway in human spermatozoa that is mediated by OGG1. J Cell Sci 2013;126:1488-97. [Crossref] [PubMed]
- Ribas-Maynou J, Benet J. Single and Double Strand Sperm DNA Damage: Different Reproductive Effects on Male Fertility. Genes (Basel) 2019;10:105. [Crossref] [PubMed]
- Ahmadi A, Ng SC. Fertilizing ability of DNA-damaged spermatozoa. J Exp Zool 1999;284:696-704. [Crossref] [PubMed]
- Shimura T, Inoue M, Taga M, et al. p53-dependent S-phase damage checkpoint and pronuclear cross talk in mouse zygotes with X-irradiated sperm. Mol Cell Biol 2002;22:2220-8. [Crossref] [PubMed]
- Marchetti F, Bishop J, Gingerich J, et al. Meiotic interstrand DNA damage escapes paternal repair and causes chromosomal aberrations in the zygote by maternal misrepair. Sci Rep 2015;5:7689. [Crossref] [PubMed]
- de Boer P, Ramos L, de Vries M, et al. Memoirs of an insult: sperm as a possible source of transgenerational epimutations and genetic instability. Mol Hum Reprod 2010;16:48-56. [Crossref] [PubMed]
- Klaude M, Eriksson S, Nygren J, et al. The comet assay: mechanisms and technical considerations. Mutat Res 1996;363:89-96. [Crossref] [PubMed]
- Agarwal A, Majzoub A, Baskaran S, et al. Sperm DNA Fragmentation: A New Guideline for Clinicians. World J Mens Health 2020;38:412-71. [Crossref] [PubMed]
- Ribas-Maynou J, García-Peiró A, Fernandez-Encinas A, et al. Double stranded sperm DNA breaks, measured by Comet assay, are associated with unexplained recurrent miscarriage in couples without a female factor. PLoS One 2012;7:e44679. [Crossref] [PubMed]
- Casanovas A, Ribas-Maynou J, Lara-Cerrillo S, et al. Double-stranded sperm DNA damage is a cause of delay in embryo development and can impair implantation rates. Fertil Steril 2019;111:699-707.e1. [Crossref] [PubMed]
- Muratori M, De Geyter C. Chromatin condensation, fragmentation of DNA and differences in the epigenetic signature of infertile men. Best Pract Res Clin Endocrinol Metab 2019;33:117-26. [Crossref] [PubMed]
- García-Rodríguez A, Gosálvez J, Agarwal A, et al. DNA Damage and Repair in Human Reproductive Cells. Int J Mol Sci 2018;20:31. [Crossref] [PubMed]
- Rousseaux S, Boussouar F, Gaucher J, et al. Molecular models for post-meiotic male genome reprogramming. Syst Biol Reprod Med 2011;57:50-3. [Crossref] [PubMed]
- Shaman JA, Prisztoka R, Ward WS. Topoisomerase IIB and an extracellular nuclease interact to digest sperm DNA in an apoptotic-like manner. Biol Reprod 2006;75:741-8. [Crossref] [PubMed]
- Simon L, Carrell DT. Sperm DNA damage measured by comet assay. Methods Mol Biol 2013;927:137-46. [Crossref] [PubMed]
- Enciso M, Sarasa J, Agarwal A, et al. A two-tailed Comet assay for assessing DNA damage in spermatozoa. Reprod Biomed Online 2009;18:609-16. [Crossref] [PubMed]
- De Iuliis GN, Thomson LK, Mitchell LA, et al. DNA damage in human spermatozoa is highly correlated with the efficiency of chromatin remodeling and the formation of 8-hydroxy-2'-deoxyguanosine, a marker of oxidative stress. Biol Reprod 2009;81:517-24. [Crossref] [PubMed]
- Santiso R, Tamayo M, Gosálvez J, et al. Simultaneous determination in situ of DNA fragmentation and 8-oxoguanine in human sperm. Fertil Steril 2010;93:314-8. [Crossref] [PubMed]
- Rashki Ghaleno L, Alizadeh A, Drevet JR, et al. Oxidation of Sperm DNA and Male Infertility. Antioxidants (Basel) 2021;10:97. [Crossref] [PubMed]
- Jackson SP, Bartek J. The DNA-damage response in human biology and disease. Nature 2009;461:1071-8. [Crossref] [PubMed]
- Kowalczykowski SC. Initiation of genetic recombination and recombination-dependent replication. Trends Biochem Sci 2000;25:156-65. [Crossref] [PubMed]
- Cox MM, Goodman MF, Kreuzer KN, et al. The importance of repairing stalled replication forks. Nature 2000;404:37-41. [Crossref] [PubMed]
- Takeshima T, Usui K, Mori K, et al. Oxidative stress and male infertility. Reprod Med Biol 2020;20:41-52. [Crossref] [PubMed]
- Sedha S, Kumar S, Shukla S. Role of Oxidative Stress in Male Reproductive Dysfunctions with Reference to Phthalate Compounds. Urol J 2015;12:2304-16. [PubMed]
- Hammadeh ME, Hamad MF, Montenarh M, et al. Protamine contents and P1/P2 ratio in human spermatozoa from smokers and non-smokers. Hum Reprod 2010;25:2708-20. [Crossref] [PubMed]
- Comhaire F. The role of food supplementation in the treatment of the infertile couple and for assisted reproduction. Andrologia 2010;42:331-40. [Crossref] [PubMed]
- Jensen CFS, Østergren P, Dupree JM, et al. Varicocele and male infertility. Nat Rev Urol 2017;14:523-33. [Crossref] [PubMed]
- Intasqui P, Camargo M, Del Giudice PT, et al. Sperm nuclear DNA fragmentation rate is associated with differential protein expression and enriched functions in human seminal plasma. BJU Int 2013;112:835-43. [Crossref] [PubMed]
- Zini A, Dohle G. Are varicoceles associated with increased deoxyribonucleic acid fragmentation? Fertil Steril 2011;96:1283-7. [Crossref] [PubMed]
- Wang YJ, Zhang RQ, Lin YJ, et al. Relationship between varicocele and sperm DNA damage and the effect of varicocele repair: a meta-analysis. Reprod Biomed Online 2012;25:307-14. [Crossref] [PubMed]
- Kanter M, Aktas C, Erboga M. Heat stress decreases testicular germ cell proliferation and increases apoptosis in short term: an immunohistochemical and ultrastructural study. Toxicol Ind Health 2013;29:99-113. [Crossref] [PubMed]
- Zhang MH, Shi ZD, Yu JC, et al. Scrotal heat stress causes sperm chromatin damage and cysteinyl aspartate-spicific proteinases 3 changes in fertile men. J Assist Reprod Genet 2015;32:747-55. [Crossref] [PubMed]
- Paul C, Murray AA, Spears N, et al. A single, mild, transient scrotal heat stress causes DNA damage, subfertility and impairs formation of blastocysts in mice. Reproduction 2008;136:73-84. [Crossref] [PubMed]
- Hamilton TRDS, Siqueira AFP, de Castro LS, et al. Effect of Heat Stress on Sperm DNA: Protamine Assessment in Ram Spermatozoa and Testicle. Oxid Med Cell Longev 2018;2018:5413056. [Crossref] [PubMed]
- Alshahrani S, Agarwal A, Assidi M, et al. Infertile men older than 40 years are at higher risk of sperm DNA damage. Reprod Biol Endocrinol 2014;12:103. [Crossref] [PubMed]
- Pino V, Sanz A, Valdés N, et al. The effects of aging on semen parameters and sperm DNA fragmentation. JBRA Assist Reprod 2020;24:82-6. [Crossref] [PubMed]
- Degirmenci Y, Demirdag E, Guler I, et al. Impact of the sexual abstinence period on the production of seminal reactive oxygen species in patients undergoing intrauterine insemination: A randomized trial. J Obstet Gynaecol Res 2020;46:1133-9. [Crossref] [PubMed]
- Marshburn PB, Giddings A, Causby S, et al. Influence of ejaculatory abstinence on seminal total antioxidant capacity and sperm membrane lipid peroxidation. Fertil Steril 2014;102:705-10. [Crossref] [PubMed]
- Hamada A, Esteves SC, Agarwal A. Unexplained male infertility. Hum Androl. 2011;1:2-16. [Crossref]
- Zandieh Z, Vatannejad A, Doosti M, et al. Comparing reactive oxygen species and DNA fragmentation in semen samples of unexplained infertile and healthy fertile men. Ir J Med Sci 2018;187:657-62. [Crossref] [PubMed]
- Oleszczuk K, Augustinsson L, Bayat N, et al. Prevalence of high DNA fragmentation index in male partners of unexplained infertile couples. Andrology 2013;1:357-60. [Crossref] [PubMed]
- Pelliccione F, d'Angeli A, Cinque B, et al. Activation of the immune system and sperm DNA fragmentation are associated with idiopathic oligoasthenoteratospermia in men with couple subfertility. Fertil Steril 2011;95:2676-9.e1-3.
- Li Z, Wang L, Cai J, et al. Correlation of sperm DNA damage with IVF and ICSI outcomes: a systematic review and meta-analysis. J Assist Reprod Genet 2006;23:367-76. [Crossref] [PubMed]
- Deng C, Li T, Xie Y, et al. Sperm DNA fragmentation index influences assisted reproductive technology outcome: A systematic review and meta-analysis combined with a retrospective cohort study. Andrologia 2019;51:e13263. [Crossref] [PubMed]
- Ribas-Maynou J, Yeste M, Becerra-Tomás N, et al. Clinical implications of sperm DNA damage in IVF and ICSI: updated systematic review and meta-analysis. Biol Rev Camb Philos Soc 2021;96:1284-300. [Crossref] [PubMed]
- Zini A. Are sperm chromatin and DNA defects relevant in the clinic? Syst Biol Reprod Med 2011;57:78-85. [Crossref] [PubMed]
- Chen Q, Zhao JY, Xue X, et al. The association between sperm DNA fragmentation and reproductive outcomes following intrauterine insemination, a meta analysis. Reprod Toxicol 2019;86:50-5. [Crossref] [PubMed]
- Sugihara A, Van Avermaete F, Roelant E, et al. The role of sperm DNA fragmentation testing in predicting intra-uterine insemination outcome: A systematic review and meta-analysis. Eur J Obstet Gynecol Reprod Biol 2020;244:8-15. [Crossref] [PubMed]
- Zhao J, Zhang Q, Wang Y, et al. Whether sperm deoxyribonucleic acid fragmentation has an effect on pregnancy and miscarriage after in vitro fertilization/intracytoplasmic sperm injection: a systematic review and meta-analysis. Fertil Steril 2014;102:998-1005.e8. [Crossref] [PubMed]
- Simon L, Zini A, Dyachenko A, et al. A systematic review and meta-analysis to determine the effect of sperm DNA damage on in vitro fertilization and intracytoplasmic sperm injection outcome. Asian J Androl 2017;19:80-90. [PubMed]
- Zini A, Boman JM, Belzile E, et al. Sperm DNA damage is associated with an increased risk of pregnancy loss after IVF and ICSI: systematic review and meta-analysis. Hum Reprod 2008;23:2663-8. [Crossref] [PubMed]
- Robinson L, Gallos ID, Conner SJ, et al. The effect of sperm DNA fragmentation on miscarriage rates: a systematic review and meta-analysis. Hum Reprod 2012;27:2908-17. [Crossref] [PubMed]
- Osman A, Alsomait H, Seshadri S, et al. The effect of sperm DNA fragmentation on live birth rate after IVF or ICSI: a systematic review and meta-analysis. Reprod Biomed Online 2015;30:120-7. [Crossref] [PubMed]
- McQueen DB, Zhang J, Robins JC. Sperm DNA fragmentation and recurrent pregnancy loss: a systematic review and meta-analysis. Fertil Steril 2019;112:54-60.e3. [Crossref] [PubMed]
- Tan J, Taskin O, Albert A, et al. Association between sperm DNA fragmentation and idiopathic recurrent pregnancy loss: a systematic review and meta-analysis. Reprod Biomed Online 2019;38:951-60. [Crossref] [PubMed]
- Li J, Luo L, Diao J, et al. Male sperm quality and risk of recurrent spontaneous abortion in Chinese couples: A systematic review and meta-analysis. Medicine (Baltimore) 2021;100:e24828. [Crossref] [PubMed]
- Tang L, Rao M, Yang W, et al. Predictive value of the sperm DNA fragmentation index for low or failed IVF fertilization in men with mild-to-moderate asthenozoospermia. J Gynecol Obstet Hum Reprod 2021;50:101868. [Crossref] [PubMed]
- Green KA, Patounakis G, Dougherty MP, et al. Sperm DNA fragmentation on the day of fertilization is not associated with embryologic or clinical outcomes after IVF/ICSI. J Assist Reprod Genet 2020;37:71-6. [Crossref] [PubMed]
- Bungum M, Humaidan P, Axmon A, et al. Sperm DNA integrity assessment in prediction of assisted reproduction technology outcome. Hum Reprod 2007;22:174-9. [Crossref] [PubMed]
- Oleszczuk K, Giwercman A, Bungum M. Sperm chromatin structure assay in prediction of in vitro fertilization outcome. Andrology 2016;4:290-6. [Crossref] [PubMed]
- Sun JG, Jurisicova A, Casper RF. Detection of deoxyribonucleic acid fragmentation in human sperm: correlation with fertilization in vitro. Biol Reprod 1997;56:602-7. [Crossref] [PubMed]
- Middelkamp S, van Tol HTA, Spierings DCJ, et al. Sperm DNA damage causes genomic instability in early embryonic development. Sci Adv 2020;6:eaaz7602. [Crossref] [PubMed]
- Practice Committee of the American Society for Reproductive Medicine. The clinical utility of sperm DNA integrity testing: a guideline. Fertil Steril 2013;99:673-7. [Crossref] [PubMed]
- Male Infertility Best Practice Policy Committee of the American Urological Association; Practice Committee of the American Society for Reproductive Medicine. The Optimal Evaluation of the Infertile Male: Best Pract. statement Rev. validity confirmed 2011, 2011, 1–16.
- Practice Committee of the American Society for Reproductive Medicine. Diagnostic evaluation of the infertile male: a committee opinion. Fertil Steril 2015;103:e18-25. [Crossref] [PubMed]
- ESHRE Guideline Group on RPL. ESHRE guideline: recurrent pregnancy loss. Hum Reprod Open 2018;2018:hoy004. [Crossref] [PubMed]
- Salonia A, Bettocchi C, Carvalho J, et al. European Association of Urology: EAU Guidelines on Sexual and Reproductive Health. Eur Assoc Urol 2020. Available online: https://uroweb.org/wp-content/uploads/EAU-Guidelines-on-Sexual-and-Reproductive-Health-2020.pdf
- World Health Organization. WHO laboratory manual for the examination and processing of human semen: Sixth edition. 2021.
- Cissen M, Wely MV, Scholten I, et al. Measuring Sperm DNA Fragmentation and Clinical Outcomes of Medically Assisted Reproduction: A Systematic Review and Meta-Analysis. PLoS One 2016;11:e0165125. [Crossref] [PubMed]
- Evenson DP, Kasperson K, Wixon RL. Analysis of sperm DNA fragmentation using flow cytometry and other techniques. Soc Reprod Fertil Suppl 2007;65:93-113. [PubMed]
- Sharma RK, Sabanegh E, Mahfouz R, et al. TUNEL as a test for sperm DNA damage in the evaluation of male infertility. Urology 2010;76:1380-6. [Crossref] [PubMed]
- Robbins DJ, Coleman MS. Initiator role of double stranded DNA in terminal transferase catalyzed polymerization reactions. Nucleic Acids Res 1988;16:2943-57. [Crossref] [PubMed]
- Sharma R, Masaki J, Agarwal A. Sperm DNA fragmentation analysis using the TUNEL assay. Methods Mol Biol 2013;927:121-36. [Crossref] [PubMed]
- Sharma R, Iovine C, Agarwal A, et al. TUNEL assay-Standardized method for testing sperm DNA fragmentation. Andrologia 2021;53:e13738. [Crossref] [PubMed]
- Sharma R, Ahmad G, Esteves SC, et al. Terminal deoxynucleotidyl transferase dUTP nick end labeling (TUNEL) assay using bench top flow cytometer for evaluation of sperm DNA fragmentation in fertility laboratories: protocol, reference values, and quality control. J Assist Reprod Genet 2016;33:291-300. [Crossref] [PubMed]
- Hornsby PJ, Didenko VV. In situ ligation: a decade and a half of experience. Methods Mol Biol 2011;682:49-63. [Crossref] [PubMed]
- Bungum M, Bungum L, Giwercman A. Sperm chromatin structure assay (SCSA): a tool in diagnosis and treatment of infertility. Asian J Androl 2011;13:69-75. [Crossref] [PubMed]
- Evenson DP, Larson KL, Jost LK. Sperm chromatin structure assay: its clinical use for detecting sperm DNA fragmentation in male infertility and comparisons with other techniques. J Androl 2002;23:25-43. [Crossref] [PubMed]
- Zhang Z, Zhu LL, Jiang HS, et al. Predictors of pregnancy outcome for infertile couples attending IVF and ICSI programmes. Andrologia 2016;48:874-81. [Crossref] [PubMed]
- Fernández JL, Muriel L, Rivero MT, et al. The sperm chromatin dispersion test: a simple method for the determination of sperm DNA fragmentation. J Androl 2003;24:59-66. [PubMed]
- Albert O, Reintsch WE, Chan P, et al. HT-COMET: a novel automated approach for high throughput assessment of human sperm chromatin quality. Hum Reprod 2016;31:938-46. [Crossref] [PubMed]
- Cortés-Gutiérrez EI, Fernández JL, Dávila-Rodríguez MI, et al. Two-Tailed Comet Assay (2T-Comet): Simultaneous Detection of DNA Single and Double Strand Breaks. Methods Mol Biol 2017;1560:285-93. [Crossref] [PubMed]
- Singh NP, McCoy MT, Tice RR, et al. A simple technique for quantitation of low levels of DNA damage in individual cells. Exp Cell Res 1988;175:184-91. [Crossref] [PubMed]
- Nicopoullos J, Vicens-Morton A, Lewis SEM, et al. Novel use of COMET parameters of sperm DNA damage may increase its utility to diagnose male infertility and predict live births following both IVF and ICSI. Hum Reprod 2019;34:1915-23. [Crossref] [PubMed]
- Esteves SC, Zini A, Coward RM, et al. Sperm DNA fragmentation testing: Summary evidence and clinical practice recommendations. Andrologia 2021;53:e13874. [Crossref] [PubMed]
- Agarwal A, Farkouh A, Parekh N, et al. Sperm DNA Fragmentation: A Critical Assessment of Clinical Practice Guidelines. World J Mens Health 2022;40:30-7. [Crossref] [PubMed]
- Gallegos G, Ramos B, Santiso R, et al. Sperm DNA fragmentation in infertile men with genitourinary infection by Chlamydia trachomatis and Mycoplasma. Fertil Steril 2008;90:328-34. [Crossref] [PubMed]
- Dupont C, Faure C, Sermondade N, et al. Obesity leads to higher risk of sperm DNA damage in infertile patients. Asian J Androl 2013;15:622-5. [Crossref] [PubMed]
- Sepidarkish M, Maleki-Hajiagha A, Maroufizadeh S, et al. The effect of body mass index on sperm DNA fragmentation: a systematic review and meta-analysis. Int J Obes (Lond) 2020;44:549-58. [Crossref] [PubMed]
- Zhou L, Han L, Liu M, et al. Impact of metabolic syndrome on sex hormones and reproductive function: a meta-analysis of 2923 cases and 14062 controls. Aging (Albany NY) 2020;13:1962-71. [Crossref] [PubMed]
- Mir J, Franken D, Andrabi SW, et al. Impact of weight loss on sperm DNA integrity in obese men. Andrologia 2018; [Epub ahead of print]. [Crossref] [PubMed]
- Carette C, Levy R, Eustache F, et al. Changes in total sperm count after gastric bypass and sleeve gastrectomy: the BARIASPERM prospective study. Surg Obes Relat Dis 2019;15:1271-9. [Crossref] [PubMed]
- Wood GJA, Tiseo BC, Paluello DV, et al. Bariatric Surgery Impact on Reproductive Hormones, Semen Analysis, and Sperm DNA Fragmentation in Men with Severe Obesity: Prospective Study. Obes Surg 2020;30:4840-51. [Crossref] [PubMed]
- Ni K, Steger K, Yang H, et al. A comprehensive investigation of sperm DNA damage and oxidative stress injury in infertile patients with subclinical, normozoospermic, and astheno/oligozoospermic clinical varicocoele. Andrology 2016;4:816-24. [Crossref] [PubMed]
- Abdelbaki SA, Sabry JH, Al-Adl AM, et al. The impact of coexisting sperm DNA fragmentation and seminal oxidative stress on the outcome of varicocelectomy in infertile patients: A prospective controlled study. Arab J Urol 2017;15:131-9. [Crossref] [PubMed]
- Qiu D, Shi Q, Pan L. Efficacy of varicocelectomy for sperm DNA integrity improvement: A meta-analysis. Andrologia 2021;53:e13885. [Crossref] [PubMed]
- Esteves SC, Roque M, Agarwal A. Outcome of assisted reproductive technology in men with treated and untreated varicocele: systematic review and meta-analysis. Asian J Androl 2016;18:254-8. [Crossref] [PubMed]
- Jannatifar R, Cheraghi E, Nasr-Esfahani MH, et al. Association of heat shock protein A2 expression and sperm quality after N-acetyl-cysteine supplementation in astheno-terato-zoospermic infertile men. Andrologia 2021;53:e14024. [Crossref] [PubMed]
- Alahmar AT, Sengupta P, Dutta S, et al. Coenzyme Q10, oxidative stress markers, and sperm DNA damage in men with idiopathic oligoasthenoteratospermia. Clin Exp Reprod Med 2021;48:150-5. [Crossref] [PubMed]
- Greco E, Romano S, Iacobelli M, et al. ICSI in cases of sperm DNA damage: beneficial effect of oral antioxidant treatment. Hum Reprod 2005;20:2590-4. [Crossref] [PubMed]
- Steiner AZ, Hansen KR, Barnhart KT, et al. The effect of antioxidants on male factor infertility: the Males, Antioxidants, and Infertility (MOXI) randomized clinical trial. Fertil Steril 2020;113:552-560.e3. [Crossref] [PubMed]
- Schisterman EF, Sjaarda LA, Clemons T, et al. Effect of Folic Acid and Zinc Supplementation in Men on Semen Quality and Live Birth Among Couples Undergoing Infertility Treatment: A Randomized Clinical Trial. JAMA 2020;323:35-48. [Crossref] [PubMed]
- Henkel R, Sandhu IS, Agarwal A. The excessive use of antioxidant therapy: A possible cause of male infertility? Andrologia 2019;51:e13162. [Crossref] [PubMed]
- Majzoub A, Agarwal A, Esteves SC. Antioxidants for elevated sperm DNA fragmentation: a mini review. Transl Androl Urol 2017;6:S649-53. [Crossref] [PubMed]
- Martinez M, Majzoub A. Best laboratory practices and therapeutic interventions to reduce sperm DNA damage. Andrologia 2021;53:e13736. [Crossref] [PubMed]
- Agarwal A, Gupta S, Du Plessis S, et al. Abstinence Time and Its Impact on Basic and Advanced Semen Parameters. Urology 2016;94:102-10. [Crossref] [PubMed]
- Marshburn PB, Alanis M, Matthews ML, et al. A short period of ejaculatory abstinence before intrauterine insemination is associated with higher pregnancy rates. Fertil Steril 2010;93:286-8. [Crossref] [PubMed]
- Sánchez-Martín P, Sánchez-Martín F, González-Martínez M, et al. Increased pregnancy after reduced male abstinence. Syst Biol Reprod Med 2013;59:256-60. [Crossref] [PubMed]
- Duca Y, Calogero AE, Cannarella R, et al. Current and emerging medical therapeutic agents for idiopathic male infertility. Expert Opin Pharmacother 2019;20:55-67. [Crossref] [PubMed]
- Muratori M, Baldi E. Effects of FSH on Sperm DNA Fragmentation: Review of Clinical Studies and Possible Mechanisms of Action. Front Endocrinol (Lausanne) 2018;9:734. [Crossref] [PubMed]
- Simoni M, Santi D, Negri L, et al. Treatment with human, recombinant FSH improves sperm DNA fragmentation in idiopathic infertile men depending on the FSH receptor polymorphism p.N680S: a pharmacogenetic study. Hum Reprod 2016;31:1960-9. [Crossref] [PubMed]
- Troya J, Zorrilla I. Annexin V-MACS in infertile couples as method for separation of sperm without DNA fragmentation. JBRA Assist Reprod 2015;19:66-9. [Crossref] [PubMed]
- Pacheco A, Blanco A, Bronet F, et al. Magnetic-Activated Cell Sorting (MACS): A Useful Sperm-Selection Technique in Cases of High Levels of Sperm DNA Fragmentation. J Clin Med 2020;9:3976. [Crossref] [PubMed]
- Hammoud I, Boitrelle F, Ferfouri F, et al. Selection of normal spermatozoa with a vacuole-free head (x6300) improves selection of spermatozoa with intact DNA in patients with high sperm DNA fragmentation rates. Andrologia 2013;45:163-70. [Crossref] [PubMed]
- Mangoli E, Khalili MA, Talebi AR, et al. Association between early embryo morphokinetics plus transcript levels of sperm apoptotic genes and clinical outcomes in IMSI and ICSI cycles of male factor patients. J Assist Reprod Genet 2020;37:2555-67. [Crossref] [PubMed]
- Setti AS, Braga DP, Figueira RC, et al. Intracytoplasmic morphologically selected sperm injection results in improved clinical outcomes in couples with previous ICSI failures or male factor infertility: a meta-analysis. Eur J Obstet Gynecol Reprod Biol 2014;183:96-103. [Crossref] [PubMed]
- Parmegiani L, Cognigni GE, Bernardi S, et al. "Physiologic ICSI": hyaluronic acid (HA) favors selection of spermatozoa without DNA fragmentation and with normal nucleus, resulting in improvement of embryo quality. Fertil Steril 2010;93:598-604. [Crossref] [PubMed]
- Hasanen E, Elqusi K, ElTanbouly S, et al. PICSI vs. MACS for abnormal sperm DNA fragmentation ICSI cases: a prospective randomized trial. J Assist Reprod Genet 2020;37:2605-13. [Crossref] [PubMed]
- Shirota K, Yotsumoto F, Itoh H, et al. Separation efficiency of a microfluidic sperm sorter to minimize sperm DNA damage. Fertil Steril 2016;105:315-21.e1. [Crossref] [PubMed]
- Esteves SC, Roque M, Bradley CK, et al. Reproductive outcomes of testicular versus ejaculated sperm for intracytoplasmic sperm injection among men with high levels of DNA fragmentation in semen: systematic review and meta-analysis. Fertil Steril 2017;108:456-467.e1. [Crossref] [PubMed]
- Fortunato A, Boni R, Leo R, et al. Vacuoles in sperm head are not associated with head morphology, DNA damage and reproductive success. Reprod Biomed Online 2016;32:154-61. [Crossref] [PubMed]
- Alharbi M, Hamouche F, Phillips S, et al. Use of testicular sperm in couples with SCSA-defined high sperm DNA fragmentation and failed intracytoplasmic sperm injection using ejaculated sperm. Asian J Androl 2020;22:348-53. [Crossref] [PubMed]
- Esteves SC, Roque M, Garrido N. Use of testicular sperm for intracytoplasmic sperm injection in men with high sperm DNA fragmentation: a SWOT analysis. Asian J Androl 2018;20:1-8. [Crossref] [PubMed]
- Kandil H, Agarwal A, Saleh R, et al. Editorial Commentary on Draft of World Health Organization Sixth Edition Laboratory Manual for the Examination and Processing of Human Semen. World J Mens Health 2021;39:577-80.