Inhibition of the proliferation, invasion, migration, and epithelial-mesenchymal transition of prostate cancer cells through the action of ATP1A2 on the TGF-β/Smad pathway
Introduction
Prostate cancer (PC) is a relatively common malignancy of the male genitourinary system, particularly affecting middle-aged and older men, and has a high incidence in Europe and the USA (1), with an increasing incidence in Asian countries in recent years (2). PC is highly aggressive and prone to metastasis (3), which is the cause of almost all deaths of PC patients and occurs mostly in the late stage of the disease, is usually resistant to various treatment methods and has a 5-year survival rate of >30% (4,5). Therefore, it is particularly urgent to understand the mechanism of the occurrence and development of PC and to find new and effective diagnostic techniques and treatments for PC.
The well-studied sodium pump (Na+-K+-ATPase) is widely involved in various pathological processes (6). It has different subtypes, including four different subtypes of the α subunit, which all have different functions. As an example, α1, or ATP1A1, is considered to play a tumor-promoting role in malignant gliomas, small cell lung cancer, and other tumors (7,8); α3 (ATP1A3) is considered to have the ability to promote metastasis of colon cancer (9); α4 (ATP1A4) exists only in testicular tissue (10); and repeated mutations in ATP1A2 have been found to be involved in the biological processes of lung cancer cell metabolism, division, death, and immune regulation (11). Moreover, the data of The Cancer Genome Atlas (TCGA) have demonstrated that ATP1A2 expression is markedly reduced in PC tissues, and low ATP1A2 expression in patients is associated with a lower survival rate. However, there are few published studies of the relationship between α2 (ATP1A2) and PC. Therefore, the purpose of this study was to measure the expression of ATP1A2 in PC and explore its effect on the invasion and migration of PC cells in two cell lines (PC-3 and DU-145), with the goal of expanding our knowledge of the role of Na+-K+-ATPase in tumors and to provide new ideas for the treatment of PC in clinical practice.
The ligand, receptor, and intracellular signal transduction molecule Smad protein of the transforming growth factor-β (TGF-β) signal transduction pathway forms a tumor-inhibitory pathway (12,13). Abnormality of any element in the pathway can cause signal transduction disorders and lead to tumorigenesis. Epithelial-mesenchymal transition (EMT) is a process in which epithelial cells lose their epithelial phenotype and acquire a stromal phenotype, which then transforms into stromal cells (14). As an important part of the deterioration and metastasis of PC, the activation of EMT is regulated by a series of gene expression changes. Previous studies have found that the TGF-β1/Smad pathway regulates the expression of Snail and Twist1 (15). An abnormally activated TGF-β1/Smad pathway mediates EMT by inducing Snail1 overexpression in most tumors (16). The binding of TGF-β1 to the TGF receptor on the cell surface leads to phosphorylation of TGF-β RII and further activates Smad3. P-Smad3 enters the nucleus to initiate the expression of Snail1 and Twist (17). Basic studies have found that the EMT of tumor cells can be reversed by inhibiting the TGF-β/Smad pathway.
In this study, we for the first time revealed the expression level and effect of ATP1A2 in PC and unraveled that ATP1A2 played significant roles in PC through the regulation of TGF-β/Smad pathway. We present the following article in accordance with the ARRIVE reporting checklist (available at https://tau.amegroups.com/article/view/10.21037/tau-21-1117/rc).
Methods
Cell culture and treatment
Human PC cell lines (PC-3, VCaP, LNCaP and DU145) and human normal prostate epithelial cell line (RWPE-1) were acquired from the American Type Culture Collection (ATCC, USA). The cells were cultured in complete culture medium containing 10% fetal bovine serum (Dulbecco’s Modified Eagle Medium) at a constant temperature of 37 °C in a 5% CO2 incubator. Cells in the logarithmic growth phase and in good condition were selected for the experiment.
The constructs pcDNA-ATP1A2 and si-ATP1A2 and their negative controls were transiently transfected into PC-3 and DU145 cells using Lipofectamine® 3000 Reagent (Invitrogen, USA) according to the manufacturer’s instructions. The transfected PC-3 and DU145 cells were divided into five groups: (I) pcDNA-NC group (treated with pcDNA-NC), (II) pcDNA-ATP1A2 group (treated with pcDNA-ATP1A2), (III) si-NC group (treated with ATP1A2 siRNA negative control), (IV) si-ATP1A2 group (treated with ATP1A2 siRNA) and (V) si-ATP1A2 + LY364947 group (treated with ATP1A2 siRNA and LY364947). LY364947, an inhibitor of the TGF-β/Smad pathway, was obtained from Abcam, UK. All cells were then cultured in a 37 °C incubator for 48 h. pcDNA-NC, pcDNA-ATP1A2, si-NC, and si-ATP1A2 were supplied by GenePharma (Shanghai, China).
Quantitative real-time PCR (qRT-PCR)
qRT-PCR was performed to analyze ATP1A2 expression as previously described (18,19). Total RNA from cells was extracted using TRIzol reagent. After concentration and purity detection, cDNA was synthesized with FirstStrand cDNA Synthesis Supermix (Transgen Biotech, Beijing, China) according to the manufacturer’s instructions. The expression of ATP1A2 was determined through qRT-PCR using an ABI 7500 system (Applied Biosystems, USA), with Green qPCR Supermix (Transgen Biotech, Beijing, China). The gene expression levels were calculated using the 2-ΔΔCt method. GAPDH was used as an internal reference. The primers were supplied by GenePharma as follows:
ATP1A2 (sense): 5'-GGGCACAGATATGGTCCCTG-3', (antisense): 5'-TTGTCCGTCTGGGAGTTTCG-3'; GAPDH (sense): 5'-TTAGGAAAGCCTGCCGGTGA-3', (antisense): 5'-GCCCAATACGACCAAATCAGAGAAT-3'.
Colony formation
Cells in the logarithmic growth phase were inoculated into 6-well plates according to appropriate cell density, and were evenly dispersed and cultured in a cell incubator for 2 weeks. When cell colonies were observable in the well plate with the naked eye, the cells were fixed with methanol, stained with crystal violet, and counted directly.
EdU detection
Cells were seeded in a 96-well plate at a density of 5×103 cells/well and 200 µL/well. After the cells fused, they were incubated with 50 mmol/L EdU solution (Beyotime, Nantong, China) for 2 h, fixed with 4% formaldehyde and treated with 0.5% Triton for 20 min to permeabilize at room temperature. Staining of the cell nuclei was performed using 4',6-diamidino-2-phenylindole (DAPI; Sigma, USA). The prepared samples were photographed under a fluorescence microscope.
Cell apoptosis
The apoptotic ability of PC-3 and DU145 cells was analyzed using the Annexin-V-FITC cell apoptosis assay kit (Beyotime) following the manufacturer’s protocols. After being resuspended in the binding buffer, the transfected PC-3 and DU145 cells were stained with Annexin V-FITC and propidium iodide in the dark for 15 min. The cells were then analyzed with a flow cytometer (FACScan, BD Bioscience, USA).
Transwell assay
After cells were digested and centrifuged, 800 µL of culture medium containing 10% serum was added to the lower chamber of transwell chambers (Corning, Corning, NY, USA), 100 µL cell suspension was added to the upper chamber, and the mixtures were cultured for 24 h in an incubator at 37 °C. The chamber was then removed to allow the fluid in the upper chamber to dry out, the cells was fixed with 4% paraformaldehyde at room temperature for 30 min, and finally stained with crystal violet at room temperature for 30 min. The chamber was then gently rinsed with clean water and photographed under a microscope.
Cell invasion assay was firstly carried out using a Corning Polycarbonate Membrane Insert transwell chamber (8-µm pore size, Corning, USA) coated with Matrigel (BD Bioscience). The subsequent steps were as described above.
Western blot
Western blot was performed as previously described (20,21). Cell proteins were extracted with RIPA lysis buffer (KeyGene Biotech, Nanjing, China), and the protein concentration was determined by BCA (KeyGene Biotech). After SDS-PAGE electrophoresis and membrane transfer, the membranes were blocked with 5% non-fat milk for 1 h and then incubated with primary antibody overnight at 4 °C. After incubation with horseradish peroxidase-conjugated secondary antibodies at room temperature for 1 h, the membranes were visualized using enhanced chemiluminescence reagents (Beyotime). The primary antibodies used in the study included Bax, caspase-3, Bcl-2, E-cadherin, matrix metalloproteinase (MMP)-2, MMP-9, and β-actin (purchased from ProteinTech, IL, USA), anti-Snail, anti-vimentin, anti-N-cadherin, anti-β-catenin, anti-TGF-β, α-SMA, anti-p-Smad2, anti-Smad2, anti-p-Smad3, anti-Smad3, and anti-cleaved caspase-3 were purchased from Cell Signaling Technology (MA, USA). Anti-ATP1A2 was supplied by Abcam (Cambridge, UK).
In vivo xenograft model
Ten male BALB/c nude mice (4 weeks old), obtained from Beijing Vital River, were injected subcutaneously in their right dorsal flanks with 1×107 DU145 cells transfected with either pcDNA-NC or pcDNA-ATP1A2. Animal experiments were performed under a project license (No. KYKT2018-043-A1) granted by the Animal Ethics Committee of Affiliated Dongguan People’s Hospital, Southern Medical University, in compliance with Affiliated Dongguan People’s Hospital, Southern Medical University guidelines for the care and use of animals. Subcutaneous tumor volumes were measured every 6 days and calculated as: volume = length × width2 × 1/2. On the 30th day after injection, the mice were killed humanely and the subcutaneous tumors were removed and weighed.
Bioinformatic analysis
The differential expression of ATP1A2 in PC and normal tissues, and the prognostic analysis of PC patients were performed by Gene Expression Profiling Interactive Analysis (GEPIA; http://gepia2.cancer-pku.cn) based on TCGA (https://cancergenome.nih.gov/).
Statistical analysis
The data were analyzed using SPSS 21.0, and the measurement data were expressed as mean ± standard deviation (SD). Statistical analysis was performed using Student’s t-test or one-way analysis of variance (ANOVA) test. The difference was considered statistically significant at P<0.05.
Results
ATP1A2 expression in PC tissues and cells
The results of GEPIA based on TCGA database revealed that ATP1A2 expression is markedly reduced in PC tissues (Figure 1A), and low ATP1A2 expression in patients is associated with a lower survival rate (Figure 1B). Moreover in our study, the mRNA and protein expressions of ATP1A2 were significantly reduced in PC cells compared with normal human prostate epithelial cells (Figure 1C,1D). Compared with the other PC cells, PC-3 and DU145 cells showed the lowest ATP1A2 expression, which is why we selected them for the subsequent experiments.
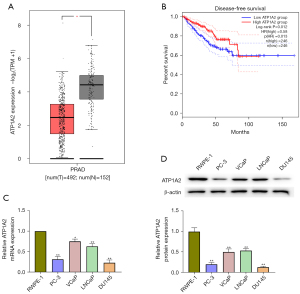
Effect of ATP1A2 on PC cell proliferation
We transfected PC-3 and DU145 cells with pcDNA-ATP1A2 and si-ATP1A2 to evaluate the role of ATP1A2 in cell proliferation. The mRNA and protein expressions of ATP1A2 increased after transfection with pcDNA-ATP1A2, but decreased after transfection with si-ATP1A2 (Figure 2A,2B). As shown in Figure 2C, ATP1A2 overexpression reduced the number of PC-3 and DU145 cell clones. In contrast, ATP1A2 silencing increased the colony number (Figure 2C). EdU staining also showed that proliferation of PC-3 and DU145 cells was inhibited by ATP1A2 overexpression and promoted by ATP1A2 silencing (Figure 2D). These data suggested that ATP1A2 markedly inhibited the proliferation of PC cells.
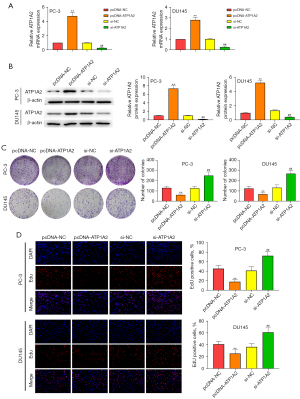
Effect of ATP1A2 on apoptosis of PC-3 and DU145 cells
Overexpression and silencing of ATP1A2 promoted or inhibited apoptosis in PC-3 and DU145 cells, respectively (Figure 3A). Western blot results revealed that overexpression of ATP1A2 elevated the expression of Bax and cleaved caspase-3, and reduced the expression of Bcl-2 in PC-3 and DU145 cells, whereas ATP1A2 silencing had the opposite effect (Figure 3B). These data demonstrated that ATP1A2 promoted apoptosis of PC cells.
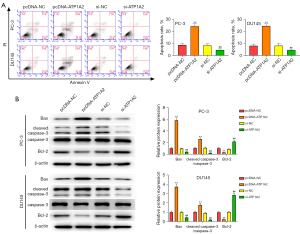
Effect of ATP1A2 on the migration and invasion of PC cells
Inhibition of tumor cell migration and invasion can effectively inhibit tumor metastasis. Overexpression and silencing of ATP1A2 inhibited or promoted the migration and invasion of PC cells, respectively (Figure 4A,4B). Overexpression of ATP1A2 significantly inhibited the expression of MMP-2 and MMP-9, whereas silencing of ATP1A2 significantly promoted their expression (Figure 4C).
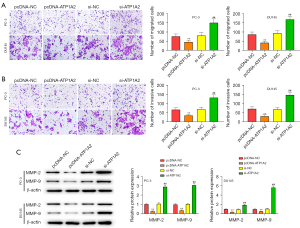
Effect of ATP1A2 on EMT in PC cells
Subsequently, we detected the expression of EMT-related marker proteins. Overexpression of ATP1A2 significantly inhibited Snail, α-SMA, vimentin, N-cadherin, and β-catenin expression and promoted E-cadherin expression, whereas silencing of ATP1A2 had the opposite result (Figure 5A,5B). These data revealed that ATP1A2 could significantly inhibit EMT in PC cells.
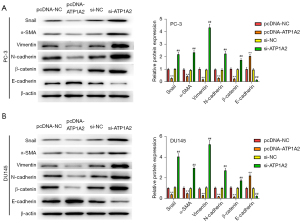
Effect of ATP1A2 on the TGF-β/Smad pathway in PC cells
The TGF-β-Smad signaling pathway plays a key role in cellular EMT, which can reduce the adhesion between cells, allowing tumor cells to penetrate the basement membrane, extensively invade adjacent tissues, and develop distant metastases. Therefore, we examined the effect of ATP1A2 on the TGF-β/Smad pathway. As shown in Figure 6A,6B, overexpression of ATP1A2 markedly inhibited the expression of TGF-β, p-Smad2, and p-Smad3 in PC-3 and DU145 cells, whereas ATP1A2 silencing significantly promoted their expression. These data confirmed that ATP1A2 inhibited the activation of the TGF-β/Smad pathway.
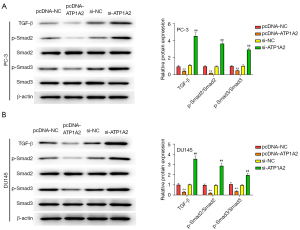
Effect of ATP1A2 inhibiting the TGF-β/Smad pathway
As seen in Figure 7A, the facilitating effect of ATP1A2 silencing on the expression of TGF-β, p-Smad2, and p-Smad3 in PC-3 and DU145 cells was significantly weakened by LY364947 (an inhibitor of the TGF-β/Smad pathway). The effect of ATP1A2 silencing on DU145 cell proliferation was reversed by LY364947 (Figure 7B), whereas the inhibitory effect of ATP1A2 silencing on the apoptosis of DU145 cells was reversed by LY364947 (Figure 7C). Moreover, LY364947 reversed the effect of ATP1A2 silencing on the migration and invasion of DU145 cells (Figure 7D). These results suggested that ATP1A2 can suppress the proliferation, migration and invasion of PC cells, and promote their apoptosis by inhibiting the TGF-β/Smad pathway.
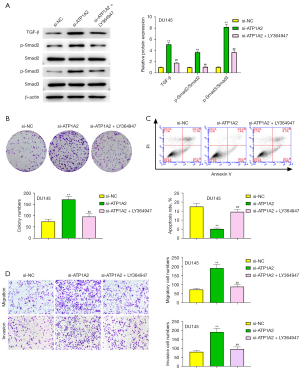
Effect of ATP1A2 on tumor growth in vivo
To explore the function of ATP1A2 on tumor growth in vivo, the xenograft assay was performed (Figure 8A). Tumor volume and weight were significantly decreased in the pcDNA-ATP1A2 group when compared with the pcDNA-NC group (Figure 8B,8C). These results revealed that ATP1A2 overexpression can inhibit tumor growth in vivo.
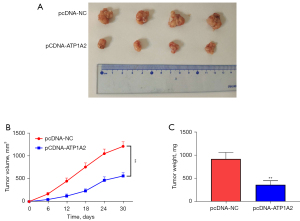
Discussion
The symptoms of early PC are subtle, and the disease is difficult to diagnosis until it has progressed (22). Although some patients improve after treatment, many others progress to resistant PC, which leads to difficulties in treatment and poor prognosis; consequently the patient’s quality of life is seriously affected (23,24). Therefore, finding more effective therapeutic targets is urgent.
As the target protein of cardiotonic glycosides, Na+-K+-ATPase has been widely studied in the context of heart failure, arrhythmia, and other diseases; its signal transduction function has been gradually discovered and recognized by researchers, and its role in tumorigenesis is now a major focus (25). ATP1A2 is mainly expressed in the adult heart, vascular smooth muscle, skeletal muscle, brain, adipocytes, cartilage, and bone, but less attention has been given to ATP1A2 in tumors because of its tissue specificity (26,27). In this study, we revealed that ATP1A2 is expressed at low levels in PC tissues and cells, and that PC patients with low ATP1A2 expression have a higher mortality rate. Therefore, we speculate that ATP1A2 plays a regulatory role in PC. The expression of ATP1A2 was lowest in PC-3 and DU145 cells compared with other PC cell lines, so we used them for further analyses. Previous studies have revealed that the proliferation, apoptosis, invasion and metastasis of tumor cells are the pathological basis of the development of cancers (28,29). In this study, the results showed that ATP1A2 significantly inhibited the proliferation, migration and invasion of PC-3 and DU145 cells and promoted the apoptosis of PC-3 and DU145 cells.
EMT is a necessary process for the distant metastasis of PC (28). MMPs such as MMP-2 and MMP-9 degrade the extracellular matrix (ECM) and enhance cell invasion (30). Our results confirmed that ATP1A2 overexpression inhibited MMP-2 and MMP-9 expression. Snail significantly regulates the EMT process, which is closely related to the loss of E-cadherin and upregulation of vimentin and fibronectin (31-33). Cytological studies have confirmed that reduced intracellular stability of the Snail protein can inhibit the EMT process and the malignant phenotype of cancer cells (34). In this study, we confirmed that ATP1A2 can significantly inhibit EMT-associated proteins and Snail in PC cells.
TGF-β1 is highly expressed in a variety of tumors, and its role in tumor development has been explored both in vitro and in transgenic mice (35). However, the role of TGF-β1 in carcinogenesis is complex. Many tumor cells become insensitive to TGF-β1-mediated growth inhibition, suggesting that increased TGF-β1 expression may alter the tumor microenvironment, such as inducing local angiogenesis, ECM generation, and inhibiting immune surveillance, which provides a favorable environment for the proliferation of tumor cells (36). In the process of tumor development, the increase in TGF-β1 synthesis plays a role in promoting tumor development (37). Malignant proliferation of cancer cells originating from the prostate epithelium lose cell polarity after EMT, resulting in decreased intercellular adhesion and enhanced potential for invasion and migration. At the same time, the expression of epithelial markers E-cadherin and tight junction protein ZO-1 is downregulated, and the expression of stromal cell markers N-cadherin and vimentin is upregulated (38,39). TGF-β is an effective inducer of EMT, which is characterized by decreased expression of E-cadherin, and upregulation of interstitial proteins (40). During cancer progression, classical Smad is a key influential factor for TGF-β-induced EMT, which is abnormally reactivated during cancer progression and can be used to regulate EMT through the interaction of related transcription factors (41). TGF-β can induce EMT by activating Smad3 and Smad2 signaling (42). In this study, we confirmed that ATP1A2 significantly inhibited the activation of the TGF-β/Smad pathway in PC. Moreover, the rescue experiments further directly verified that the TGF-β/Smad pathway was a target of ATP1A2. All these results confirmed that the promotion of ATP1A2 could be choosed for PC treatment in the future.
However, there are also shortcomings in our research. There is not only one signaling pathway that has a potential targeting relationship with ATP1A2. Therefore, whether ATP1A2 affects other pathways in PC will be further investigated in our future experiments. More work is required to explore how ATP1A2 can be used as a novel therapeutic target for the treatment of PC.
Conclusions
In summary, we first confirmed that the expression of ATP1A2 was decreased in PC tissues and cells. We also revealed that ATP1A2 significantly inhibits the proliferation, migration, invasion and EMT of PC cells, and may be regulated through the TGF-β/Smad pathway. ATP1A2 may be an effective therapeutic target for the treatment of PC.
Acknowledgments
Funding: None.
Footnote
Reporting Checklist: The authors have completed the ARRIVE reporting checklist. Available at https://tau.amegroups.com/article/view/10.21037/tau-21-1117/rc
Data Sharing Statement: Available at https://tau.amegroups.com/article/view/10.21037/tau-21-1117/dss
Conflicts of Interest: All authors have completed the ICMJE uniform disclosure form (available at https://tau.amegroups.com/article/view/10.21037/tau-21-1117/coif). The authors have no conflicts of interest to declare.
Ethical Statement: The authors are accountable for all aspects of the work in ensuring that questions related to the accuracy or integrity of any part of the work are appropriately investigated and resolved. Animal experiments were performed under a project license (No. KYKT2018-043-A1) granted by the Animal Ethics Committee of Affiliated Dongguan People’s Hospital, Southern Medical University, in compliance with Affiliated Dongguan People’s Hospital, Southern Medical University guidelines for the care and use of animals. A protocol was prepared before the study without registration.
Open Access Statement: This is an Open Access article distributed in accordance with the Creative Commons Attribution-NonCommercial-NoDerivs 4.0 International License (CC BY-NC-ND 4.0), which permits the non-commercial replication and distribution of the article with the strict proviso that no changes or edits are made and the original work is properly cited (including links to both the formal publication through the relevant DOI and the license). See: https://creativecommons.org/licenses/by-nc-nd/4.0/.
References
- Teoh JYC, Hirai HW, Ho JMW, et al. Global incidence of prostate cancer in developing and developed countries with changing age structures. PLoS One 2019;14:e0221775. [Crossref] [PubMed]
- Kimura T, Egawa S. Epidemiology of prostate cancer in Asian countries. Int J Urol 2018;25:524-31. [Crossref] [PubMed]
- Wang G, Zhao D, Spring DJ, et al. Genetics and biology of prostate cancer. Genes Dev 2018;32:1105-40. [Crossref] [PubMed]
- Lin D. Commentary on "The evolutionary history of lethal metastatic prostate cancer." Gundem G, Van Loo P, Kremeyer B, Alexandrov LB, Tubio JM, Papaemmanuil E, Brewer DS, Kallio HM, Högnäs G, Annala M, Kivinummi K, Goody V, Latimer C, O'Meara S, Dawson KJ, Isaacs W, Emmert-Buck MR, Nykter M, Foster C, Kote-Jarai Z, Easton D, Whitaker HC, ICGC Prostate UK Group, Neal DE, Cooper CS, Eeles RA, Visakorpi T, Campbell PJ, McDermott U, Wedge DC, Bova GS, University of Washington-Urology, Seattle, WA. Nature 2015; 520(7547):353-7. Urol Oncol 2016;34:520-1. [Crossref] [PubMed]
- Siegel RL, Miller KD, Jemal A. Cancer Statistics, 2017. CA Cancer J Clin 2017;67:7-30. [Crossref] [PubMed]
- Lingrel JB. The physiological significance of the cardiotonic steroid/ouabain-binding site of the Na,K-ATPase. Annu Rev Physiol 2010;72:395-412. [Crossref] [PubMed]
- Ramos-Alvarez I, Lee L, Jensen RT. Cyclic AMP-dependent protein kinase A and EPAC mediate VIP and secretin stimulation of PAK4 and activation of Na+,K+-ATPase in pancreatic acinar cells. Am J Physiol Gastrointest Liver Physiol 2019;316:G263-77. [Crossref] [PubMed]
- Xu ZW, Wang FM, Gao MJ, et al. Targeting the Na(+)/K(+)-ATPase alpha1 subunit of hepatoma HepG2 cell line to induce apoptosis and cell cycle arresting. Biol Pharm Bull 2010;33:743-51. [Crossref] [PubMed]
- Baker Bechmann M, Rotoli D, Morales M, et al. Na,K-ATPase Isozymes in Colorectal Cancer and Liver Metastases. Front Physiol 2016;7:9. [Crossref] [PubMed]
- Lingrel JB, Kuntzweiler T. Na+,K(+)-ATPase. J Biol Chem 1994;269:19659-62. [Crossref] [PubMed]
- Asiedu MK, Thomas CF Jr, Dong J, et al. Pathways Impacted by Genomic Alterations in Pulmonary Carcinoid Tumors. Clin Cancer Res 2018;24:1691-704. [Crossref] [PubMed]
- Xu J, Shao T, Song M, et al. MIR22HG acts as a tumor suppressor via TGFβ/SMAD signaling and facilitates immunotherapy in colorectal cancer. Mol Cancer 2020;19:51. [Crossref] [PubMed]
- Sakai S, Ohhata T, Kitagawa K, et al. Long Noncoding RNA ELIT-1 Acts as a Smad3 Cofactor to Facilitate TGFβ/Smad Signaling and Promote Epithelial-Mesenchymal Transition. Cancer Res 2019;79:2821-38. [Crossref] [PubMed]
- Voon DC, Huang RY, Jackson RA, et al. The EMT spectrum and therapeutic opportunities. Mol Oncol 2017;11:878-91. [Crossref] [PubMed]
- Gupta P, Srivastava SK. HER2 mediated de novo production of TGFβ leads to SNAIL driven epithelial-to-mesenchymal transition and metastasis of breast cancer. Mol Oncol 2014;8:1532-47. [Crossref] [PubMed]
- Zhang H, von Gise A, Liu Q, et al. Yap1 is required for endothelial to mesenchymal transition of the atrioventricular cushion. J Biol Chem 2014;289:18681-92. [Crossref] [PubMed]
- Xian S, Chen A, Wu X, et al. Activation of activin/Smad2 and 3 signaling pathway and the potential involvement of endothelial-mesenchymal transition in the valvular damage due to rheumatic heart disease. Mol Med Rep 2021;23:10. [PubMed]
- Zhao Y, Balasubramanian B, Guo Y, et al. Dietary Enteromorpha Polysaccharides Supplementation Improves Breast Muscle Yield and Is Associated With Modification of mRNA Transcriptome in Broiler Chickens. Front Vet Sci 2021;8:663988. [Crossref] [PubMed]
- Liu WC, Guo Y, Zhao ZH, et al. Algae-Derived Polysaccharides Promote Growth Performance by Improving Antioxidant Capacity and Intestinal Barrier Function in Broiler Chickens. Front Vet Sci 2020;7:601336. [Crossref] [PubMed]
- Guo Y, Balasubramanian B, Zhao ZH, et al. Marine algal polysaccharides alleviate aflatoxin B1-induced bursa of Fabricius injury by regulating redox and apoptotic signaling pathway in broilers. Poult Sci 2021;100:844-57. [Crossref] [PubMed]
- Liu WC, Ou BH, Liang ZL, et al. Algae-derived polysaccharides supplementation ameliorates heat stress-induced impairment of bursa of Fabricius via modulating NF-κB signaling pathway in broilers. Poult Sci 2021;100:101139. [Crossref] [PubMed]
- Rycaj K, Li H, Zhou J, et al. Cellular determinants and microenvironmental regulation of prostate cancer metastasis. Semin Cancer Biol 2017;44:83-97. [Crossref] [PubMed]
- Carbonetti G, Wilpshaar T, Kroonen J, et al. FABP5 coordinates lipid signaling that promotes prostate cancer metastasis. Sci Rep 2019;9:18944. [Crossref] [PubMed]
- Padhani AR, Lecouvet FE, Tunariu N, et al. METastasis Reporting and Data System for Prostate Cancer: Practical Guidelines for Acquisition, Interpretation, and Reporting of Whole-body Magnetic Resonance Imaging-based Evaluations of Multiorgan Involvement in Advanced Prostate Cancer. Eur Urol 2017;71:81-92. [Crossref] [PubMed]
- Witkowski J. The influence of the membrane sodium pump activity on the ability of human peripheral blood lymphocytes to form early rosettes with SRBC. Immunology 1985;55:339-45. [PubMed]
- Matchkov VV, Krivoi II. Specialized Functional Diversity and Interactions of the Na,K-ATPase. Front Physiol 2016;7:179. [Crossref] [PubMed]
- Kutz LC, Mukherji ST, Wang X, et al. Isoform-specific role of Na/K-ATPase α1 in skeletal muscle. Am J Physiol Endocrinol Metab 2018;314:E620-9. [Crossref] [PubMed]
- Hu W, Yan F, Ru Y, et al. MIIP inhibits EMT and cell invasion in prostate cancer through miR-181a/b-5p-KLF17 axis. Am J Cancer Res 2020;10:630-47. [PubMed]
- Wu X, Xiao Y, Zhou Y, et al. LncRNA FOXP4-AS1 is activated by PAX5 and promotes the growth of prostate cancer by sequestering miR-3184-5p to upregulate FOXP4. Cell Death Dis 2019;10:472. [Crossref] [PubMed]
- Kumar S, Das A, Barai A, et al. MMP Secretion Rate and Inter-invadopodia Spacing Collectively Govern Cancer Invasiveness. Biophys J 2018;114:650-62. [Crossref] [PubMed]
- Lin X, Chai G, Wu Y, et al. RNA m6A methylation regulates the epithelial mesenchymal transition of cancer cells and translation of Snail. Nat Commun 2019;10:2065. [Crossref] [PubMed]
- Xiong W, Zhang L, Liu H, et al. E2 -mediated EMT by activation of β-catenin/Snail signalling during the development of ovarian endometriosis. J Cell Mol Med 2019;23:8035-45. [Crossref] [PubMed]
- Taki M, Abiko K, Baba T, et al. Snail promotes ovarian cancer progression by recruiting myeloid-derived suppressor cells via CXCR2 ligand upregulation. Nat Commun 2018;9:1685. [Crossref] [PubMed]
- Jang D, Kwon H, Choi M, et al. Sumoylation of Flotillin-1 promotes EMT in metastatic prostate cancer by suppressing Snail degradation. Oncogene 2019;38:3248-60. [Crossref] [PubMed]
- Yang L, Pang Y, Moses HL. TGF-beta and immune cells: an important regulatory axis in the tumor microenvironment and progression. Trends Immunol 2010;31:220-7. [Crossref] [PubMed]
- Itatani Y, Kawada K, Sakai Y. Transforming Growth Factor-β Signaling Pathway in Colorectal Cancer and Its Tumor Microenvironment. Int J Mol Sci 2019;20:5822. [Crossref] [PubMed]
- Haque S, Morris JC. Transforming growth factor-β: A therapeutic target for cancer. Hum Vaccin Immunother 2017;13:1741-50. [Crossref] [PubMed]
- De Craene B, Berx G. Regulatory networks defining EMT during cancer initiation and progression. Nat Rev Cancer 2013;13:97-110. [Crossref] [PubMed]
- Singh A, Settleman J. EMT, cancer stem cells and drug resistance: an emerging axis of evil in the war on cancer. Oncogene 2010;29:4741-51. [Crossref] [PubMed]
- Zhang L, Wang X, Lai C, et al. PMEPA1 induces EMT via a non-canonical TGF-β signalling in colorectal cancer. J Cell Mol Med 2019;23:3603-15. [Crossref] [PubMed]
- Yao Y, Chen R, Wang G, et al. Exosomes derived from mesenchymal stem cells reverse EMT via TGF-β1/Smad pathway and promote repair of damaged endometrium. Stem Cell Res Ther 2019;10:225. [Crossref] [PubMed]
- Fenizia C, Bottino C, Corbetta S, et al. SMYD3 promotes the epithelial-mesenchymal transition in breast cancer. Nucleic Acids Res 2019;47:1278-93. [Crossref] [PubMed]