High dose-rate brachytherapy in the treatment of prostate cancer
Introduction
Patients diagnosed with localized prostate cancer face many treatment options which may include active surveillance, radical prostatectomy, external beam radiotherapy (EBRT), brachytherapy, androgen deprivation therapy or various combinations of the above. The choice of therapy depends on factors such as risk grouping group, bulk of disease, presence of obstructive urinary symptoms, prostate volume, patient age and co-morbidities, patient preference and treatment availability. For patients being treated with radiation, brachytherapy has an important role to play, either as monotherapy or as a boost in combination with external beam radiation. Brachytherapy also has a role as salvage treatment in patients who develop local recurrence following previous EBRT.
Either permanent seed low dose-rate (LDR) or high dose-rate (HDR) brachytherapy provide a highly conformal escalation of dose to the cancer, with greater sparing of surrounding normal organs than that achievable with any form of EBRT. In a dosimetric study by Georg and colleagues, radiation dose to normal tissues including rectal and bladder wall was significantly lower with brachytherapy (either HDR or permanent seeds) compared to external techniques of volumetric-modulated arc therapy (VMAT), intensity modulated proton therapy or scanned carbon-ion therapy (1). The lowest dose to normal tissues was obtained with HDR. Spratt and colleagues drew a similar conclusion comparing HDR dosimetry with that of stereotactic body radiotherapy (SBRT)—HDR delivers a higher dose within the prostate and lower dose to adjacent organs at risk (2).
HDR is defined as a dose delivered at a rate >12 Gy/hour, although usually much higher, often in excess of 1 Gy per minute. This rapid dose delivery results in radiobiological effects similar to that of extremely hypofractionated EBRT such as stereotactic body radiotherapy (SBRT), and is thought to be selectively more damaging to cells with low alpha/beta ratios such as late responding normal tissues or prostate cancer. Dose is delivered using a high activity radioactive source that is attached to a cable and driven along implanted catheters. Source physical size varies from 3.5 to 5 mm in length with a diameter of 0.5 to 1 mm, depending on the particular equipment. Step size can range between 1 and 5 mm, but a step size of 2–3 mm is most commonly used. Iridium-192 (average photon energy 380 keV) and Cobalt-60 (Photon energies 1.17 and 1.33 Mev) are the two most commonly used sources, and can produce similar dose distributions within the treated volume.
Rationale
HDR brachytherapy has been used to treat prostate cancer since the 1980’s (3). Much of the original rationale was to overcome the problem of suboptimal dosimetry seen with LDR implants due to seed loss or misplacement (4). Because HDR planning occurs with the catheters in place, there is greater certainty that the delivered dose is the same as the planned dose. HDR brachytherapy results in more consistent dosimetry than that seen with LDR, with less variability in target coverage and lower relative dose to urethra, bladder and rectum (5,6). Dosing outside the prostate to cover extraprostatic extension of disease or seminal vesicle invasion is more reliably achieved with HDR. Brachytherapy dose distributions are inherently heterogeneous with high areas of dose around the sources. Because of the ability to readily vary where within the prostate the higher dose is delivered, HDR is more amenable to intraprostatic dose painting and focal treatments than LDR.
Radiation dose is rapidly delivered over a matter of minutes, rather than slowly over weeks or months as with LDR. Dynamic changes in prostate volume due to edema following implantation will therefore have little effect on the HDR dose received. The very different duration of treatment delivery between HDR and LDR results in quicker resolution of urinary symptoms following HDR (7). The short treatment time also provides a possible advantage in using HDR to treat more rapidly dividing cancers than LDR. It is likely that the mean alpha/beta ratio for prostate cancer is very low, possibly even less than 1.0 (8). This is lower than the alpha/beta ratio of adjacent normal tissues, and would suggest that prostate cancer cells are more sensitive to radiation delivered in large fraction size than are normal late responding cells. This provides further radiobiological rationale for using HDR brachytherapy as a means of biological dose escalation to the cancer without exceeding tolerance of adjacent late responding normal tissues.
Clinical indications
HDR brachytherapy is indicated as a boost in combination with EBRT, as monotherapy, and as a salvage option following previous EBRT. Just as with LDR brachytherapy, patients being considered for HDR brachytherapy should have no medical contraindications to undergoing anesthesia, with the following relative contra-indications:
- An obstructive prostate resulting in an International Prostate Symptom Score (IPSS) greater than 18, restrictive flow pattern or residual urine volume >100 cc;
- A median lobe protruding >1 cm into the bladder;
- Prostate volume >60 cc;
- Previous trans-urethral resection of prostate (TURP);
- Active inflammatory bowel disease;
- Previous pelvic radiation treatment.
There are few absolute contraindications to undergoing HDR brachytherapy, and in each situation the extra risks of toxicity associated with the above need to be considered in the light of other treatment options and patient preference. For example, while patients who have had a previous TURP or large prostate volume are excluded from most brachytherapy clinical trial protocols, many experienced practitioners will often still treat these patients with HDR, sometimes with minor modifications of technique with excellent results (9). Similarly, for patients with inflammatory bowel disease, brachytherapy results in less rectal dose than EBRT so may be the preferred treatment technique. In situations where patients have had previous pelvic radiation it may be desirable to avoid further radiation, yet HDR can be used as a salvage following previous radical prostate radiotherapy. Pubic arch obstruction is often discussed but is rarely, if ever, a contra-indication in practice, as minor changes to the insertion technique or adjustment of patient position invariably allows satisfactory catheter placement.
HDR boost
When used as a boost, brachytherapy delivers a high intraprostatic dose, while the external beam component supplements dose within the prostate and also treats potential extraprostatic extension of disease. The 2017 American Society of Clinical Oncology (ASCO)/Cancer Care Ontario (CCO) brachytherapy guidelines state that brachytherapy boost (either LDR or HDR) should be offered to eligible intermediate and high risk patients choosing radiation treatment (10). This recommendation is based on the results of three randomized controlled trials demonstrating improved disease-free survival in patients treated with brachytherapy combined with external beam compared to those treated with external beam alone. Each of these clinical trials used a different form of brachytherapy boost. Sathya and colleagues performed an early randomized trial comparing a boost using LDR iridium-192 to EBRT alone in patients with locally advanced prostate cancer (11,12). Patients randomized to receive 40 Gy EBRT and a 35 Gy brachytherapy boost had a biochemical failure rate of 29% compared to a failure rate of 61% in those randomized to receive 66 Gy with EBRT alone. Although the EBRT dose is low by modern standards, the study confirmed the principle that brachytherapy boost in combination with moderate dose EBRT resulted in higher cancer control rates than that achievable with EBRT alone. Hoskin and colleagues completed a randomized trial of HDR boost in a cohort of patients with mostly intermediate and high risk disease (13). Patients were randomized to receive either EBRT alone to a dose of 55 Gy in 20 fractions, or HDR boost (17 Gy/2 fractions) combined with EBRT to a dose of 35.75 Gy in 13 fractions. Those in the HDR boost arm had a 31% reduction in risk of recurrence. Late urinary toxicity was the same in both treatment arms. The EBRT dose would also be considered low by contemporary standards, with an equivalent dose of approximately 68 Gy at standard fractionation. In the ASCENDE-RT clinical trial, 398 patients with intermediate (31%) and high risk (69%) disease were randomized to receive either EBRT to a dose of 78 Gy in 39 fractions or 46 Gy EBRT combined with an LDR boost using iodine-125 to 115 Gy (14). Brachytherapy boost reduced the risk of recurrence by over 50%—the recurrence rate at 9 years was 38% vs. 17% in the EBRT and brachytherapy boost arms, respectively.
There are no completed randomized trial comparing oncologic outcomes of HDR boost with modern dose escalated EBRT. The Canadian Clinical Trials Group (CCTG) has completed a randomized phase II clinical trial of HDR boost (15 Gy single fraction HDR and 37.5 Gy/15 fractions EBRT) and image guided EBRT (choice of 78 Gy/39 fractions or 60 Gy/20 fractions). Treatment was well tolerated acutely in both arms, but slow accrual and new guidelines will make it difficult to proceed to a larger Phase III clinical trial.
In addition to the above randomized data, there is an abundance of non-comparative series reporting favorable outcome with HDR boost (15). As can be seen from Table 1, there are many mature single center series, including over 6,000 patients and with a median follow-up of up to 10 years (16-40). There is significant variability in the brachytherapy dose and fractionation used in the different series, but disease-free survival is consistently high—approximately 95% for low risk, 91% for intermediate risk and 82% for high risk. Furthermore, rates of toxicity are low. Late grade 3 rectal toxicity is rare and the rate of late grade 3 urinary toxicity is most commonly <5%, although rates as high as 14% have been reported. The most common late grade 3 urinary toxicity is stricture, which seems to be dependent on technique and era of treatment.
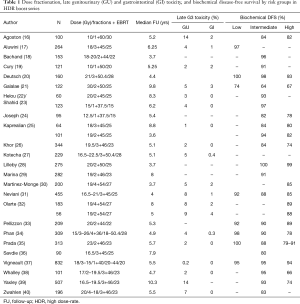
Full table
Population data has been reported to support the use of HDR boost in intermediate and high-risk disease. Ishiyama and colleagues conducted a retrospective review of 3,242 patients treated with HDR boost from 16 Asian hospitals (41). Five and 10-year biochemical control rates were 90.6% and 81.4%, respectively, in a population where 67% were in a high or very high-risk grouping.
Non-randomized comparative data is available. Spratt and colleagues reported outcome data on 870 consecutive patients with intermediate risk prostate treated with either IMRT alone to 86.4 Gy, or 50.4 Gy IMRT with a brachytherapy boost—either permanent seed or HDR (42). With a median follow-up of 5.3 years, both the biochemical disease-free survival and distant metastases-free survival rates were significantly higher in the brachytherapy boost patients at 92% vs. 81%, and 97% vs. 93% for brachytherapy boost and IMRT, respectively. Late genitourinary toxicity rates were similar in both arms, suggesting that the improved clinical outcomes were obtained without an increase in side-effects.
Kishan and colleagues reported a multi-institution comparative analysis of patients with Gleason 9–10 prostate cancer treated with either radical prostatectomy, EBRT or HDR boost (43). The authors reported significantly improved distant metastases-free survival rates with HDR boost (90%) compared with EBRT (67%) or radical prostatectomy (62%) at 10 years. Updated data including over 1,400 patients has further reported an improved prostate cancer-specific mortality with HDR boost compared to the other two treatments with less use of salvage and systemic treatments (44). The data also suggests that these favorable outcomes can be achieved with shorter duration of adjuvant androgen deprivation.
In summary, HDR brachytherapy boost results in a high disease control rates for men with localized prostate cancer, with strong evidence that it provides improved cancer control rates compared to EBRT alone without a significant increase in toxicity.
Dose and fractionation for HDR boost
The American Brachytherapy Society consensus guidelines for HDR prostate brachytherapy was unable to recommend a particular dose fractionation schedule for HDR boost, reporting high biochemical control rates despite a wide variation in dose and fractionation (45). Recommendations from the European brachytherapy group, GEC/ESTRO reference the following published EBRT dose fractionation schedules (46):
- 45 Gy in 25 fractions over 5 weeks;
- 46 Gy in 23 fractions over 4.5 weeks;
- 35.7 Gy in 13 fractions over 3 weeks;
- 37.5 Gy in 15 fractions over 3 weeks.
Combined with the following HDR brachytherapy schedules:
- 15 Gy in 3 fractions;
- 11–22 Gy in 2 fractions;
- 12–15 Gy in 1 fraction.
The dose and fractionation should be safe, effective, and efficient. There was initial concern about safety of large dose per fraction. Early reports used 4 fractions of 3–4 Gy each (4), but as more data emerged, this was replaced with regimens using a smaller number of larger fractions. Martinez and colleagues increased HDR dose in sequential cohorts from 3 fractions of 5.5 Gy to 2 fractions of 11.5 Gy, with an improved disease-free survival seen for those treated with two fractions of 9.5 Gy, or higher (47). A dose of 9.5 Gy × 2 in combination with an external beam dose of 45 Gy in 25 fractions was chosen for the RTOG 0321 clinical trial, and shown to be safe with a late grade 3 toxicity rate of 2.5% (48). Morton and colleagues investigated a yet more hypofractionated regimen of single fraction 15 Gy HDR in combination with 37.5 Gy delivered in 15 fractions over 3 weeks. This protocol began as a Phase II clinical trial for patients with intermediate risk prostate cancer in 2005 (49). At a median follow-up of 6.2 years, the 5-year biochemical disease-free survival was over 97%, with a median nadir PSA of 0.08 ng/mL (22), and a positive re-biopsy rate of under 2% (50). Late grade 3 toxicity rate was 4% (23). Clinical efficacy and toxicity were no different from the previously used regimen of HDR delivered in two fractions of 10 Gy with an external beam dose of 45 Gy in 25 fractions. Single fraction HDR boost is safe and makes efficient use of resources. It has been widely adopted as the preferred fractionation schedule, with evidence of equivalence with previously used multi-fraction regimens (24,37,51). A single 15 Gy has become the standard HDR boost dose in current Radiation Therapy Oncology Group clinical trials (RTOG 0924 and RTOG 1115).
Focal boosting with increased dose to areas of gross disease is readily achieved with HDR. Most reports use multimodality fusion, where the planning image sets (CT or ultrasound) are co-registered with a diagnostic multiparametric MRI. This can involve either a rigid or elastic co-registration. The purpose of this strategy can either be to improve local control by dose escalation to the gross disease, and/or to reduce toxicity by selective dose de-escalation to the remainder or the gland. Such approaches are well tolerated, but it is not yet known how they compare with more conventional whole gland treatments (52,53).
HDR monotherapy
The ASCO/CCO brachytherapy guidelines indicate that LDR brachytherapy as monotherapy may be offered to patients with low and low-intermediate risk prostate cancer (10). This is partly based on the results of the RTOG 0232 randomized trial which showed that LDR monotherapy was as effective as LDR combined with EBRT, but with less toxicity (54). Low-intermediate risk patients are considered to be those with Gleason 7 and PSA <10 ng/mL, or Gleason 6 and PSA between 10 and 20 ng/mL. The authors felt that there was insufficient data to recommend either for or against use of HDR monotherapy in this population. The rationale for HDR monotherapy is similar to the rationale for LDR monotherapy: in this population of patients, the risk of significant extraprostatic disease is low and brachytherapy alone should be able to encompass the full extent of disease. The main advantage of HDR in this setting is the more rapid resolution of acute urinary symptoms, which typically persist for 6 or more months following an LDR implant as dose is slowly delivered. Martinez and colleagues has shown less acute dysuria, frequency and urgency with HDR monotherapy compared to LDR (7).
The optimal dose and fractionation for HDR monotherapy remains unclear. As can be seen from Table 2, a wide range of dose and fractionation has been reported. Out of concern for potential late toxicity of large dose per fraction, many of the earlier series used many small fractions, often 6 or more (55). Mature series of 6-fraction regimens using 6.5–7.5 Gy per fraction report long term biochemical control rates of over 90% for patients with intermediate risk disease, with long term toxicity rates of under 5%, which is very comparable to that seen with LDR monotherapy (56-59). Similar data with long median follow-up is available to support using four fractions of 9.5 Gy (60). Given the logistic challenges and resource implications of delivering multiple fractions, several investigators have sought to reduce the number of fractions needed. Based on linear-quadratic predictions with an alpha/beta of 1.5, dose fractionations of 11.5 Gy × 3, 13.5 Gy × 2 or 19 Gy × 1 would deliver biologically equivalent doses of 260–280 Gy, theoretically equivalent to external beam doses of 110–120 Gy at 2 Gy per fraction. As can be seen in Table 2, favorable biochemical control rates of over 90% have been reported from series using 2- and 3-fraction protocols (61-65). The largest series using a 3-fraction regimen was reported by Strouthos and colleagues (62), which included a total of 450 patients treated to a dose of 34.5 Gy, each fraction separated by 21 days. Most of the patients had intermediate (30%) or high risk (26%) disease, and 13% also received adjuvant androgen deprivation. Less than 1% experienced late complications. The biochemical control was remarkably good at 96% for low and intermediate risk patients and 92% for high risk, results almost identical to those from the same institution with a dose of 38 Gy in 4 fractions (60).
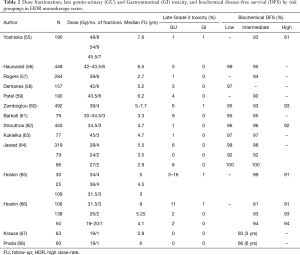
Full table
Results of single fraction HDR monotherapy have also been reported, with conflicting efficacy data. Krauss and colleagues reported a 3-year biochemical disease-free survival following single fraction 19 Gy of 93% in 58 patients, at a median follow-up of 2.9 years (67). Hoskin and colleagues reported a 4-year biochemical disease-free survival of 94% following single fraction HDR (66). A dose of 19 Gy was used in 23 patients, and 20 Gy in 26. Median follow-up was 4.1 years, and remarkably 43% had high risk disease. Three-quarters of patients also received adjuvant androgen deprivation therapy. The control rate was found to be no different than that of historical cohorts treated with 2 fractions of 13 Gy or 3 fractions of 10.5 Gy. The experience of Prada and colleagues was quite different—when data was first reported at a median follow-up time of 19 months the disease-free survival was over 90%, but with a longer median follow-up of 6 years the biochemical disease-free survival fell to only 66% (68). Of concern, 92% of these patients had Gleason 6 prostate cancer and one-third also received adjuvant androgen deprivation.
Morton and colleagues have completed a randomized trial comparing single fraction 19 Gy to two fractions of 13.5 Gy in patients with low and intermediate risk disease (69). Both treatment regimens were very well tolerated with an acute retention rate of 2.4% and a grade 3 toxicity rate of <1%. Less urinary symptoms and grade 2 erectile dysfunction was found in the single fraction arm (11.5% vs. 29%) at 12 months. PSA decline was not as rapid following single 19 Gy, however, and median PSA was significantly higher at 3 years—1.45 ng/mL following single 19 Gy and 0.48 ng/mL following 13.5 Gy × 2 (70). Local recurrence has been seen only in the single fraction arm. In contrast to other single fraction HDR series, no patient received adjuvant androgen deprivation therapy. This raises concern that linear quadratic extrapolations may overestimate the biological effectiveness of large single fraction treatments.
The low rate of toxicity seen following single fraction 19 Gy suggests that further dose escalation may be safe. One strategy is to increase the dose to the whole prostate, but HDR readily allows for intraprostatic dose painting and selective escalation of dose to areas of gross disease within the prostate. An ongoing clinical trial of the Canadian Clinical Trials Group (clinicaltrials.gov NCT02960087) randomizes men with low and low-intermediate risk prostate cancer to monotherapy with either single fraction HDR or an LDR implant using iodine-125. The HDR involves treating the entire prostate to 19 Gy, but with a simultaneous boost to dominant lesions within the prostate identified on multiparametric magnetic resonance imaging (mpMRI). The primary outcome measure is prostate cancer control rate at 4 years with HDR and LDR.
In summary, HDR monotherapy delivered in multiple fractions is a well tolerated and effective treatment for men with low and low-intermediate risk disease. Several authors also report excellent outcome in high risk disease. The optimal dose and fractionation remains undefined. A monotherapy dose of 27 Gy in two fractions appears effective and well tolerated. Although single fraction HDR monotherapy is an attractive option and associated with very little toxicity, long-term efficacy remains to be established, particularly without use of adjuvant androgen deprivation.
Salvage HDR
Biochemical failure occurs in 20–50% of patients following EBRT. Of those with a rising PSA undergoing prostate biopsy, 65% will be found to have viable cancer, usually at the site of initial disease (71). Local cancer recurrence is thought to pose a risk for further development of distant metastasis. Local salvage treatment could potentially prevent symptoms from local progression of disease and also prevent further distant metastases.
Salvage prostatectomy is associated with a subsequent recurrence-free survival ranging from 30–80%, but with a significant risk of incontinence, anastomotic stricture and rectal injury. Other salvage options include local ablative treatments such as high-intensity focused ultrasound (HIFU) or cryotherapy, and brachytherapy. Whole gland LDR salvage has been reported by several investigators, with a similar range of efficacy to that reported following radical prostatectomy. Grade 3 urinary and rectal toxicity has been reported in up to 30% and 20% of cases, respectively. The rate of grade 3 late urinary toxicity following salvage whole gland LDR was 14% in the RTOG 0526 clinical trial (72).
Salvage HDR is also an option in this patient population, with comparable outcomes to LDR (73), and with possible advantages due to its unique radiobiology and ease of dose conformality. Whole prostate HDR salvage has been reported by several authors using different dose and fractionation schemes. Chen and colleagues reported a 51% disease-free survival in a series of 52 patients treated to a dose of 36 Gy in 6 fractions (74). Yamada and colleagues reported a disease-free survival of 69% in 42 patients treated to 32 Gy in 4 fractions (75). Wojcieszek and colleagues reported a 67% disease-free survival among 83 patients treated to 30 Gy in 3 fractions (76). Jiang and colleagues also used a dose of 30 Gy in 3 fractions, reporting a 5-year disease-free survival of 45% in 22 patients (77). Three of the series reported late grade 3 urinary toxicity rates of 1–3%, and one a rate of 13%.
A particular advantage of HDR is the ability to deliver focal treatments within the prostate. As up to 95% of recurrences within the prostate occur at the site of initial dominant disease (78,79), ongoing research has investigated focal salvage approaches guided by mpMRI or functional imaging. Chung and colleagues have completed a clinical trial of ultrafocal HDR boost to the recurrent nodule in 15 patients, delivering a dose of 27 Gy in 2 fractions. At a median follow-up of 30 months, the biochemical disease-free survival was 71% (80). Toxicity was minimal, with local ablation of treated nodule seen in 10 of 12 patients undergoing repeat mpMRI. Relapse elsewhere in the prostate was apparent in 25%, and an ongoing clinical trial is investigating a strategy of focal boosting while treating the remainder of the prostate to a lower dose (NCT02560181).
HDR technique
Key steps for HDR brachytherapy are:
- Catheter placement under image guidance, usually TRUS;
- Imaging with catheters in place: TRUS, CT or MRI;
- Contouring of target(s), organs at risk and catheter reconstruction on planning system. This step may also include multimodality image co-registration to delineate gross disease;
- Dwell time optimization;
- Quality assurance;
- Treatment delivery.
The particulars of the technique will depend on availability of resources including imaging, anesthesia and shielded room. Either general or spinal anesthesia can be used. Single fraction treatments are generally delivered on an out-patient basis, with patients discharged home shortly after the procedure, while multi-fraction treatments either require admission with catheters in place for one or more days, or else re-insertion of catheters for each treatment.
Whatever technique is used, the first step involves catheter insertion, almost always under TRUS guidance (Figure 1). For whole gland treatment, 16 catheters are commonly used, with 12 catheters around the periphery and 4 more centrally placed. This distribution of catheters allows good prostate coverage, while keeping urethral and rectal dose low. When focal boosting to a dominant nodule is planned, one or two additional catheters within the nodule will help focal dose escalation (Figure 2).
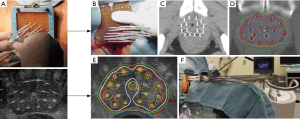
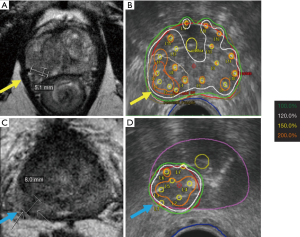
The next step is to acquire images for planning, ideally with the same patient positioning and geometry as will be used during treatment delivery. For TRUS-based planning, the patient is usually treated while still under anesthesia with the TRUS probe still in place, guaranteeing that no catheter displacement or change in anatomy will occur (81). When CT-planning is used, the template is fixed to the perineum, the legs brought down from the lithotomy position, and the patient transferred to a CT scanner for imaging. Care must be taken to have a process in place to avoid catheter displacement during patient transfers and changes in patient positioning, as catheter movement can lead to reduced prostate coverage and increased dose to urethra and penile bulb (82).
Images are transferred to a planning system on which the clinical target volume—usually the prostate, and organs at risk—urethra, rectum and often bladder—are contoured. No further expansion is needed for the planning target volume (PTV) which is the same as the clinical target volume. On occasion, a dominant nodule or gross tumor volume is contoured for boosting. This is usually defined in advance on an mpMRI, and some form of coregistration with the planning images is performed. This can involve rigid or elastic co-registration, or sometimes a cognitive co-registration.
Once contouring has been performed and catheter position identified, dwell time optimization is performed to meet the dosimetric constraints using anatomy-based inverse planning (83). There is evidence that maintaining a high target coverage (volume receiving 100% of prescription dose, V100, or dose to 90%, D90) is important for disease control, and that dose to urethra is the most important predictor of long-term urinary morbidity (84-86). Dose to 2 cc of rectum has also been shown to be predictive of late rectal toxicity (87).
GEC-ESTRO has proposed the following dosimetric constraints for both target coverage and organs at risk (46):
- PTV V100 >95% and D90 >100%;
- Dose to 2 cc of rectum <75 Gy equivalent at 2 Gy per fraction (EQD2);
- Dose to 0.1 cc or 10% of urethra <120 Gy EQD2;
- Dose to 30% of urethra <105 EQD2.
There was insufficient data to provide recommendations on bladder or penile bulb dose limits. Considering dose to normal organs in terms of EQD2 allows the recommendations to be applied with different prescription doses, and in boost and monotherapy settings.
For a prescription dose of 15 Gy, commonly used planning objectives using real time TRUS-based planning are:
- Prostate (PTV) V100 >95%;
- Prostate V200 <14%;
- Urethra maximal dose <130% of the prescription dose;
- Urethra dose to 10% (D10) <120% of the prescription dose;
- Rectal maximal dose <15 Gy;
- Rectal volume receiving 12 Gy <0.6 cc.
Conclusions
HDR brachytherapy provides highly conformal biological dose escalation to prostate cancer. It also readily allows intraprostatic dose painting and focal treatment. Combined with external beam, HDR results in higher cancer control rates than that obtained with external beam only. Multifraction HDR as monotherapy is a well established treatment for low and intermediate risk disease, and the role of more hypofractionated and single fraction regimens is evolving. HDR is a safe and effective salvage option for patients with local failure following previous external beam treatment, with increasing interest in the role of focal salvage.
Acknowledgements
None.
Footnote
Conflicts of Interest: The authors have no conflicts of interest to declare.
References
- Georg D, Hopfgartner J, Gora J, et al. Dosimetric considerations to determine the optimal technique for localized prostate cancer among external photon, proton, or carbon-ion therapy and high-dose-rate or low-dose-rate brachytherapy. Int J Radiat Oncol Biol Phys 2014;88:715-22. [Crossref] [PubMed]
- Spratt DE, Scala LM, Folkert M, et al. A comparative dosimetric analysis of virtual stereotactic body radiotherapy to high-dose-rate monotherapy for intermediate-risk prostate cancer. Brachytherapy 2013;12:428-33. [Crossref] [PubMed]
- Bentzen JK, Ockelmann HH, Hansen HS. High Dose Rate 192Ir-Microselectron. Ugeskr Laeger 1990;152:2908-10. [PubMed]
- Mate TP, Gottesman JE, Hatton J, et al. High dose-rate afterloading 192Iridium prostate brachytherapy: feasibility report. Int J Radiat Oncol Biol Phys 1998;41:525-33. [Crossref] [PubMed]
- Wang Y, Sankreacha R, Al-Hebshi A, et al. Comparative study of dosimetry between high-dose-rate and permanent prostate implant brachytherapies in patients with prostate adenocarcinoma. Brachytherapy 2006;5:251-5. [Crossref] [PubMed]
- Major T, Polgár C, Jorgo K, et al. Dosimetric comparison between treatment plans of patients treated with low-dose-rate vs. high-dose-rate interstitial prostate brachytherapy as monotherapy: Initial findings of a randomized clinical trial. Brachytherapy 2017;16:608-15. [Crossref] [PubMed]
- Martinez AA, Demanes J, Vargas C, et al. High-dose-rate prostate brachytherapy: an excellent accelerated-hypofractionated treatment for favorable prostate cancer. Am J Clin Oncol 2010;33:481-8. [Crossref] [PubMed]
- Vogelius IR, Bentzen SM. Meta-analysis of the alpha/beta ratio for prostate cancer in the presence of an overall time factor: bad news, good news, or no news? Int J Radiat Oncol Biol Phys 2013;85:89-94. [Crossref] [PubMed]
- Luo HL, Fang FM, Kang CH, et al. Can high-dose-rate brachytherapy prevent the major genitourinary complication better than external beam radiation alone for patients with previous transurethral resection of prostate? Int Urol Nephrol 2013;45:113-9. [Crossref] [PubMed]
- Chin J, Rumble RB, Kollmeier M, et al. Brachytherapy for Patients With Prostate Cancer: American Society of Clinical Oncology/Cancer Care Ontario Joint Guideline Update. J Clin Oncol 2017;35:1737-43. [Crossref] [PubMed]
- Sathya JR, Davis IR, Julian JA, et al. Randomized trial comparing iridium implant plus external-beam radiation therapy with external-beam radiation therapy alone in node-negative locally advanced cancer of the prostate. J Clin Oncol 2005;23:1192-9. [Crossref] [PubMed]
- Dayes IS, Parpia S, Gilbert J, et al. Long-term results of a randomized trial comparing iridium implant plus external beam radiation therapy with external beam radiation therapy alone in node-negative locally advanced cancer of the prostate. Int J Radiat Oncol Biol Phys 2017;99:90-3. [Crossref] [PubMed]
- Hoskin PJ, Rojas AM, Bownes PJ, et al. Randomised trial of external beam radiotherapy alone or combined with high-dose-rate brachytherapy boost for localised prostate cancer. Radiother Oncol 2012;103:217-22. [Crossref] [PubMed]
- Morris WJ, Tyldesley S, Rodda S, et al. Androgen Suppression Combined with Elective Nodal and Dose Escalated Radiation Therapy (the ASCENDE-RT Trial): an analysis of survival endpoints for a randomized trial comparing a low-dose-rate brachytherapy boost to a dose-escalated external beam boost for high- and intermediate-risk prostate cancer. Int J Radiat Oncol Biol Phys 2017;98:275-85. [Crossref] [PubMed]
- Morton GC. High-dose-rate brachytherapy boost for prostate cancer: rationale and technique. J Contemp Brachytherapy 2014;6:323-30. [Crossref] [PubMed]
- Agoston P, Major T, Frohlich G, et al. Moderate dose escalation with single-fraction high-dose-rate brachytherapy boost for clinically localized intermediate- and high-risk prostate cancer: 5-year outcome of the first 100 consecutively treated patients. Brachytherapy 2011;10:376-84. [Crossref] [PubMed]
- Aluwini S, van Rooij PH, Kirkels WJ, et al. High-dose-rate brachytherapy and external-beam radiotherapy for hormone-naive low- and intermediate-risk prostate cancer: a 7-year experience. Int J Radiat Oncol Biol Phys 2012;83:1480-5. [Crossref] [PubMed]
- Bachand F, Martin AG, Beaulieu L, et al. An eight-year experience of HDR brachytherapy boost for localized prostate cancer: biopsy and PSA outcome. Int J Radiat Oncol Biol Phys 2009;73:679-84. [Crossref] [PubMed]
- Cury FL, Duclos M, Aprikian A, et al. Single-fraction high-dose-rate brachytherapy and hypofractionated external beam radiation therapy in the treatment of intermediate-risk prostate cancer - long term results. Int J Radiat Oncol Biol Phys 2012;82:1417-23. [Crossref] [PubMed]
- Deutsch I, Zelefsky MJ, Zhang Z, et al. Comparison of PSA relapse-free survival in patients treated with ultra-high-dose IMRT versus combination HDR brachytherapy and IMRT. Brachytherapy 2010;9:313-8. [Crossref] [PubMed]
- Galalae RM, Zakikhany NH, Geiger F, et al. The 15-year outcomes of high-dose-rate brachytherapy for radical dose escalation in patients with prostate cancer-A benchmark for high-tech external beam radiotherapy alone? Brachytherapy 2014;13:117-22. [Crossref] [PubMed]
- Helou J, D’Alimonte L, Loblaw A, et al. High dose-rate brachytherapy boost for intermediate risk prostate cancer: Long-term outcomes of two different treatment schedules and early biochemical predictors of success. Radiother Oncol 2015;115:84-9. [Crossref] [PubMed]
- Shahid N, Loblaw A, Chung HT, et al. Long-term toxicity and health-related quality of life after single-fraction high dose rate brachytherapy boost and hypofractionated external beam radiotherapy for intermediate-risk prostate cancer. Clin Oncol (R Coll Radiol) 2017;29:412-20. [Crossref] [PubMed]
- Joseph N, Taylor C, O’Hara C, et al. A combined single high-dose rate brachytherapy boost with hypofractionated external beam radiotherapy results in a high rate of biochemical disease free survival in localised intermediate and high risk prostate cancer patients. Radiother Oncol 2016;121:299-303. [Crossref] [PubMed]
- Kaprealian T, Weinberg V, Speight JL, et al. High-dose-rate brachytherapy boost for prostate cancer: comparison of two different fractionation schemes. Int J Radiat Oncol Biol Phys 2012;82:222-7. [Crossref] [PubMed]
- Khor R, Duchesne G, Tai KH, et al. Direct 2-arm comparison shows benefit of high-dose-rate brachytherapy boost vs external beam radiation therapy alone for prostate cancer. Int J Radiat Oncol Biol Phys 2013;85:679-85. [Crossref] [PubMed]
- Kotecha R, Yamada Y, Pei X, et al. Clinical outcomes of high-dose-rate brachytherapy and external beam radiotherapy in the management of clinically localized prostate cancer. Brachytherapy 2013;12:44-9. [Crossref] [PubMed]
- Lilleby W, Tafjord G, Raabe NK. Implementation of high-dose-rate brachytherapy and androgen deprivation in patients with prostate cancer. Int J Radiat Oncol Biol Phys 2012;83:933-9. [Crossref] [PubMed]
- Marina O, Gustafson GS, Kestin LL, et al. Comparison of dose-escalated, image-guided radiotherapy vs. dose-escalated, high-dose-rate brachytherapy boost in a modern cohort of intermediate-risk prostate cancer patients. Brachytherapy 2014;13:59-67. [Crossref] [PubMed]
- Martinez-Monge R, Moreno M, Ciervide R, et al. External-beam radiation therapy and high-dose rate brachytherapy combined with long-term androgen deprivation therapy in high and very high prostate cancer: preliminary data on clinical outcome. Int J Radiat Oncol Biol Phys 2012;82:e469-76. [Crossref] [PubMed]
- Neviani CB, Miziara MA, de Andrade Carvalho H. Results of high dose-rate brachytherapy boost before 2D or 3D external beam irradiation for prostate cancer. Radiother Oncol 2011;98:169-74. [Crossref] [PubMed]
- Olarte A, Cambeiro M, Moreno-Jimenez M, et al. Dose escalation with external beam radiation therapy and high-dose-rate brachytherapy combined with long-term androgen deprivation therapy in high and very high risk prostate cancer: Comparison of two consecutive high-dose-rate schemes. Brachytherapy 2016;15:127-35. [Crossref] [PubMed]
- Pellizzon AC, Salvajoli J, Novaes P, et al. Updated results of high-dose rate brachytherapy and external beam radiotherapy for locally and locally advanced prostate cancer using the RTOG-ASTRO Phoenix definition. Int Braz J Urol 2008;34:293-301. [Crossref] [PubMed]
- Phan TP, Syed AM, Puthawala A, et al. High dose rate brachytherapy as a boost for the treatment of localized prostate cancer. J Urol 2007;177:123-7; discussion 127. [Crossref] [PubMed]
- Prada PJ, Gonzalez H, Fernandez J, et al. Biochemical outcome after high-dose-rate intensity modulated brachytherapy with external beam radiotherapy: 12 years of experience. BJU Int 2012;109:1787-93. [Crossref] [PubMed]
- Savdie R, Symons J, Spernat D, et al. High-dose rate brachytherapy compared with open radical prostatectomy for the treatment of high-risk prostate cancer: 10 year biochemical freedom from relapse. BJU Int 2012;110 Suppl 4:71-6. [Crossref] [PubMed]
- Vigneault E, Mbodji K, Magnan S, et al. High-dose-rate brachytherapy boost for prostate cancer treatment: Different combinations of hypofractionated regimens and clinical outcomes. Radiother Oncol 2017;124:49-55. [Crossref] [PubMed]
- Whalley D, Patanjali N, Jackson M, et al. HDR brachytherapy combined with external beam radiation for localised prostate cancer: early experience from the Sydney Cancer Centre. J Med Imaging Radiat Oncol 2012;56:220-6. [Crossref] [PubMed]
- Yaxley JW, Lah K, Yaxley JP, et al. Long-term outcomes of high-dose-rate brachytherapy for intermediate- and high-risk prostate cancer with a median follow-up of 10 years. BJU Int 2017;120:56-60. [Crossref] [PubMed]
- Zwahlen DR, Andrianopoulos N, Matheson B, et al. High-dose-rate brachytherapy in combination with conformal external beam radiotherapy in the treatment of prostate cancer. Brachytherapy 2010;9:27-35. [Crossref] [PubMed]
- Ishiyama H, Kamitani N, Kawamura H, et al. Nationwide multi-institutional retrospective analysis of high-dose-rate brachytherapy combined with external beam radiotherapy for localized prostate cancer: An Asian Prostate HDR-BT Consortium. Brachytherapy 2017;16:503-10. [Crossref] [PubMed]
- Spratt DE, Zumsteg ZS, Ghadjar P, et al. Comparison of high-dose (86.4 Gy) IMRT vs combined brachytherapy plus IMRT for intermediate-risk prostate cancer. BJU Int 2014;114:360-7. [PubMed]
- Kishan AU, Shaikh T, Wang PC, et al. Clinical outcomes for patients with gleason score 9-10 prostate adenocarcinoma treated with radiotherapy or radical prostatectomy: a multi-institutional comparative analysis. Eur Urol 2017;71:766-73. [Crossref] [PubMed]
- Kishan AU, Ciezki JP, Ross AE, et al. Extremely dose escalated radiation therapy improves cancer-specific survival compared with radical prostatectomy or conventionally dose-escalated radiation therapy in gleason score 9-10 prostate adenocarcinoma: a multi-institutional analysis of 1403 patients. Int J Radiat Oncol Biol Phys 2017;96:s131. [Crossref]
- Yamada Y, Rogers L, Demanes DJ, et al. American Brachytherapy Society consensus guidelines for high-dose-rate prostate brachytherapy. Brachytherapy 2012;11:20-32. [Crossref] [PubMed]
- Hoskin PJ, Colombo A, Henry A, et al. GEC/ESTRO recommendations on high dose rate afterloading brachytherapy for localised prostate cancer: an update. Radiother Oncol 2013;107:325-32. [Crossref] [PubMed]
- Martinez AA, Gonzalez J, Ye H, et al. Dose escalation improves cancer-related events at 10 years for intermediate- and high-risk prostate cancer patients treated with hypofractionated high-dose-rate boost and external beam radiotherapy. Int J Radiat Oncol Biol Phys 2011;79:363-70. [Crossref] [PubMed]
- Hsu IC, Bae K, Shinohara K, et al. Phase II trial of combined high-dose-rate brachytherapy and external beam radiotherapy for adenocarcinoma of the prostate: preliminary results of RTOG 0321. Int J Radiat Oncol Biol Phys 2010;78:751-8. [Crossref] [PubMed]
- Morton G, Loblaw A, Cheung P, et al. Is single fraction 15Gy the preferred high dose-rate brachytherapy boost dose for prostate cancer? Radiother Oncol 2011;100:463-7. [Crossref] [PubMed]
- D’Alimonte L, Helou J, Sherman C, et al. The clinical significance of persistent cancer cells on prostate biopsy after high-dose-rate brachytherapy boost for intermediate-risk prostate cancer. Brachytherapy 2015;14:309-14. [Crossref] [PubMed]
- Falk AT, Demontoy S, Chamorey E, et al. High-dose-rate brachytherapy boost for prostate cancer: Comparison of three different fractionation schemes. Brachytherapy 2017;16:993-9. [Crossref] [PubMed]
- Vigneault E, Mbodji K, Racine LG, et al. Image-guided high-dose-rate brachytherapy boost to the dominant intraprostatic lesion using multiparametric magnetic resonance imaging including spectroscopy: Results of a prospective study. Brachytherapy 2016;15:746-51. [Crossref] [PubMed]
- Gomez-Iturriaga A, Casquero F, Urresola A, et al. Dose escalation to dominant intraprostatic lesions with MRI-transrectal ultrasound fusion High-Dose-Rate prostate brachytherapy. Prospective phase II trial. Radiother Oncol 2016;119:91-96. [Crossref] [PubMed]
- Phase A. 3 Study comparing combined external beam radiation and transperineal interstitial permanent brachytherapy with brachytherapy alone for selected patients with intermediate-risk prostatic Carcinoma. Int J Radiat Oncol Biol Phys 2016;96:S4. BR P, Winter K, Sanda MG, et al. Initial Report of NRG Oncology/RTOG 0232:. [Crossref]
- Yoshioka Y, Suzuki O, Isohashi F, et al. High-dose-rate brachytherapy as monotherapy for intermediate- and high-risk prostate cancer: clinical results for a median 8-year follow-up. Int J Radiat Oncol Biol Phys 2016;94:675-82. [Crossref] [PubMed]
- Hauswald H, Kamrava MR, Fallon JM, et al. High-dose-rate monotherapy for localized prostate cancer: 10-year results. Int J Radiat Oncol Biol Phys 2016;94:667-74. [Crossref] [PubMed]
- Rogers CL, Alder SC, Rogers RL, et al. High dose brachytherapy as monotherapy for intermediate risk prostate cancer. J Urol 2012;187:109-16. [Crossref] [PubMed]
- Demanes DJ, Martinez AA, Ghilezan M, et al. High-dose-rate monotherapy: safe and effective brachytherapy for patients with localized prostate cancer. Int J Radiat Oncol Biol Phys 2011;81:1286-92. [Crossref] [PubMed]
- Patel S, Demanes DJ, Ragab O, et al. High-dose-rate brachytherapy monotherapy without androgen deprivation therapy for intermediate-risk prostate cancer. Brachytherapy 2017;16:299-305. [Crossref] [PubMed]
- Zamboglou N, Tselis N, Baltas D, et al. High-dose-rate interstitial brachytherapy as monotherapy for clinically localized prostate cancer: treatment evolution and mature results. Int J Radiat Oncol Biol Phys 2013;85:672-8. [Crossref] [PubMed]
- Barkati M, Williams SG, Foroudi F, et al. High-dose-rate brachytherapy as a monotherapy for favorable-risk prostate cancer: a Phase II trial. Int J Radiat Oncol Biol Phys 2012;82:1889-96. [Crossref] [PubMed]
- Strouthos I, Tselis N, Chatzikonstantinou G, et al. High dose rate brachytherapy as monotherapy for localised prostate cancer. Radiother Oncol 2018;126:270-7. [Crossref] [PubMed]
- Kukielka AM, Dabrowski T, Walasek T, et al. High-dose-rate brachytherapy as a monotherapy for prostate cancer--Single-institution results of the extreme fractionation regimen. Brachytherapy 2015;14:359-65. [Crossref] [PubMed]
- Jawad MS, Dilworth JT, Gustafson GS, et al. Outcomes associated with 3 treatment schedules of high-dose-rate brachytherapy monotherapy for favorable-risk prostate cancer. Int J Radiat Oncol Biol Phys 2016;94:657-66. [Crossref] [PubMed]
- Hoskin P, Rojas A, Lowe G, et al. High-dose-rate brachytherapy alone for localized prostate cancer in patients at moderate or high risk of biochemical recurrence. Int J Radiat Oncol Biol Phys 2012;82:1376-84. [Crossref] [PubMed]
- Hoskin P, Rojas A, Ostler P, et al. Single-dose high-dose-rate brachytherapy compared to two and three fractions for locally advanced prostate cancer. Radiother Oncol 2017;124:56-60. [Crossref] [PubMed]
- Krauss DJ, Ye H, Martinez AA, et al. Favorable preliminary outcomes for men with low- and intermediate-risk prostate cancer treated with 19-Gy single-fraction high-dose-rate brachytherapy. Int J Radiat Oncol Biol Phys 2017;97:98-106. [Crossref] [PubMed]
- Prada PJ, Cardenal J, Blanco AG, et al. High-dose-rate interstitial brachytherapy as monotherapy in one fraction for the treatment of favorable stage prostate cancer: Toxicity and long-term biochemical results. Radiother Oncol 2016;119:411-6. [Crossref] [PubMed]
- Morton G, Chung HT, McGuffin M, et al. Prostate high dose-rate brachytherapy as monotherapy for low and intermediate risk prostate cancer: Early toxicity and quality-of life results from a randomized phase II clinical trial of one fraction of 19Gy or two fractions of 13.5Gy. Radiother Oncol 2017;122:87-92. [Crossref] [PubMed]
- Morton G, Chung H, McGuffin M, et al. Prostate HDR Monotherapy: initial efficacy results from a randomized trial of one versus two fractions. Brachytherapy 2017;16:s36-7. [Crossref]
- Jalloh M, Leapman MS, Cowan JE, et al. Patterns of local failure following radiation therapy for prostate cancer. J Urol 2015;194:977-82. [Crossref] [PubMed]
- Crook JM, Zhang P, Pisansky TM, et al. A prospective phase 2 trial of transperineal ultrasound-guided brachytherapy for locally recurrent prostate cancer after external beam radiation therapy (NRG/RTOG0526): initial report of late toxicity outcome. Int J Radiat Oncol Biol Phys 2017;99:s1. [Crossref]
- Kollmeier MA, McBride S, Taggar A, et al. Salvage brachytherapy for recurrent prostate cancer after definitive radiation therapy: A comparison of low-dose-rate and high-dose-rate brachytherapy and the importance of prostate-specific antigen doubling time. Brachytherapy 2017;16:1091-8. [Crossref] [PubMed]
- Chen CP, Weinberg V, Shinohara K, et al. Salvage HDR brachytherapy for recurrent prostate cancer after previous definitive radiation therapy: 5-year outcomes. Int J Radiat Oncol Biol Phys 2013;86:324-9. [Crossref] [PubMed]
- Yamada Y, Kollmeier MA, Pei X, et al. A Phase II study of salvage high-dose-rate brachytherapy for the treatment of locally recurrent prostate cancer after definitive external beam radiotherapy. Brachytherapy 2014;13:111-6. [Crossref] [PubMed]
- Wojcieszek P, Szlag M, Głowacki G, et al. Salvage high-dose-rate brachytherapy for locally recurrent prostate cancer after primary radiotherapy failure. Radiother Oncol 2016;119:405-10. [Crossref] [PubMed]
- Jiang P, van der Horst C, Kimmig B, et al. Interstitial high-dose-rate brachytherapy as salvage treatment for locally recurrent prostate cancer after definitive radiation therapy: Toxicity and 5-year outcome. Brachytherapy 2017;16:186-92. [Crossref] [PubMed]
- Chopra S, Toi A, Taback N, et al. Pathological predictors for site of local recurrence after radiotherapy for prostate cancer. Int J Radiat Oncol Biol Phys 2012;82:e441-8. [Crossref] [PubMed]
- Pucar D, Hricak H, Shukla-Dave A, et al. Clinically significant prostate cancer local recurrence after radiation therapy occurs at the site of primary tumor: magnetic resonance imaging and step-section pathology evidence. Int J Radiat Oncol Biol Phys 2007;69:62-9. [Crossref] [PubMed]
- Chung H, D’Alimonte L, Loblaw A, et al. Three-year results of a prospective study on focal salvage HDR prostate brachytherapy after previous definitive external beam radiotherapy. Brachytherapy 2017;16:s63. [Crossref]
- Morton GC. Prostate high-dose-rate brachytherapy: transrectal ultrasound based planning, a technical note. Pract Radiat Oncol 2015;5:238-40. [Crossref] [PubMed]
- Holly R, Morton GC, Sankreacha R, et al. Use of cone-beam imaging to correct for catheter displacement in high dose-rate prostate brachytherapy. Brachytherapy 2011;10:299-305. [Crossref] [PubMed]
- Morton GC, Sankreacha R, Halina P, et al. A comparison of anatomy-based inverse planning with simulated annealing and graphical optimization for high-dose-rate prostate brachytherapy. Brachytherapy 2008;7:12-6. [Crossref] [PubMed]
- Hoskin PJ, Rojas AM, Ostler PJ, et al. Dosimetric predictors of biochemical control of prostate cancer in patients randomised to external beam radiotherapy with a boost of high dose rate brachytherapy. Radiother Oncol 2014;110:110-3. [Crossref] [PubMed]
- Hsu IC, Hunt D, Straube W, et al. Dosimetric analysis of radiation therapy oncology group 0321: The importance of urethral dose. Pract Radiat Oncol 2014;4:27-34. [Crossref] [PubMed]
- Morton GC, Loblaw DA, Chung H, et al. Health-related quality of life after single-fraction high-dose-rate brachytherapy and hypofractionated external beam radiotherapy for prostate cancer. Int J Radiat Oncol Biol Phys 2011;80:1299-305. [Crossref] [PubMed]
- Chicas-Sett R, Farga D, Perez-Calatayud MJ, et al. High-dose-rate brachytherapy boost for prostate cancer: Analysis of dose-volume histogram parameters for predicting late rectal toxicity. Brachytherapy 2017;16:511-7. [Crossref] [PubMed]